Fluorimetry Spectroscopy: Principles & Innovations
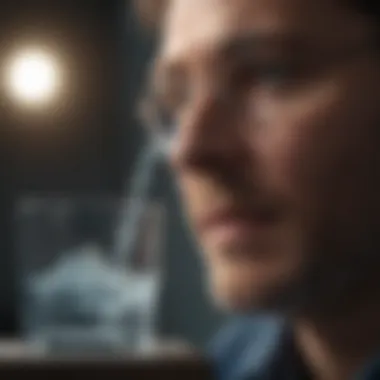
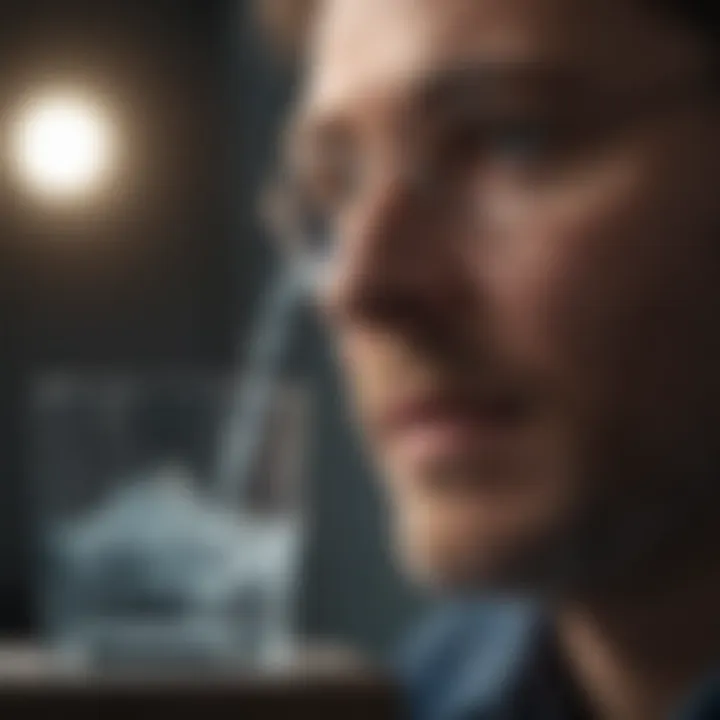
Intro
Fluorimetry spectroscopy is a powerful analytical technique used in various scientific disciplines. It leverages the principles of fluorescence to obtain information about the composition and characteristics of different substances. Fluorimetry has proven to be invaluable across fields such as environmental science, biochemistry, and material sciences. It allows researchers and professionals to analyze samples with precision and speed, making it a preferred choice for many applications.
The technique primarily involves exciting molecules with specific wavelengths of light, which then emit light at different wavelengths. This emitted light is measured, providing insights into the sample's properties. Understanding fluorimetry requires a solid grasp of both the underlying principles and the specific methodologies used in practice.
In this article, we will delve into the key concepts, current research trends, and advancements in fluorimetry spectroscopy. The aim is to foster a comprehensive understanding of how this technique operates, its relevance in ongoing research, and future directions for innovation. Through this exploration, both academics and enthusiasts will gain valuable insights into fluorimetry's role in pushing the boundaries of scientific discovery.
Intro to Fluorimetry Spectroscopy
Fluorimetry spectroscopy is a powerful analytical technique that leverages the emission of light by fluorescent substances. The importance of this field is multi-faceted, touching on various disciplines such as environmental science, biochemistry, and material science. Within this framework, understanding the core principles and applications of fluorimetry is critical for both researchers and practitioners.
Knowledge of fluorimetry allows one to accurately measure concentrations of substances, track chemical reactions, and even study dynamic biological processes. Fluorimetryβs sensitivity is one of its main benefits, enabling detection of low concentrations of analytes which might not be evident through other methods. Additionally, it's a non-destructive technique, maintaining sample integrity, which is desirable in many fields.
The relevance of fluorimetry spectroscopy stems from several key considerations. For instance, the cost-effective nature of the instrumentation needed for fluorimetry as compared to other spectroscopic methods makes it an attractive option for many laboratories. Furthermore, its capacity for high throughput is advantageous in environments where rapid results are essential.
In this introduction, we explore the essential definitions, contexts, and historical milestones that have shaped fluorimetry spectroscopy into a vital scientific tool today.
Definition and Context
Fluorimetry can be defined as the measurement of the intensity of fluorescent light emitted from a sample when excited by a light source of appropriate wavelength. This technique fundamentally relies on the phenomenon of fluorescence, where certain materials absorb light at one wavelength and subsequently emit light at another, usually longer, wavelength. The emitted light can then be quantitatively analyzed to derive significant information about the sample's composition.
Contextually, fluorimetry plays a pivotal role in various applications spanning from clinical diagnostics to environmental monitoring. As the necessity for rapid and precise analytical methods has grown, so too has the popularity of fluorimetric techniques. Many modern laboratory instruments have integrated advancements in detector technology and optics, broadening the scope of fluorimetry's applicability.
Historical Development
The historical development of fluorimetry spectroscopy traces back to the early studies of fluorescence phenomena in the 19th century. Scientists such as Sir George Gabriel Stokes, who introduced the term "fluorescence" in 1852, laid the groundwork by investigating light emission properties of certain compounds. Stokes observed that materials could emit light of longer wavelengths than that of the exciting light, a concept that remains a foundational principle in todayβs fluorometric analysis.
Advancements continued through the 20th century, with research into various fluorescent materials and their properties. The development of light sources like xenon and mercury lamps further amplified fluorimetry's capabilities. The advent of sophisticated detection technologies such as photomultiplier tubes and later, charge-coupled devices, significantly improved sensitivity and resolution.
In the latter part of the 20th century, innovations in computer technology and digital signal processing transformed data analysis methods within fluorimetry, allowing for more efficient and accurate quantitative measurements. Today, fluorimetry is not just a scientific curiosity; it is a cornerstone methodology in laboratories around the world, emphasized by its diverse applicability and critical innovations in instrumentation.
Core Principles of Fluorimetry
Understanding the core principles of fluorimetry is essential for comprehending its applications and innovations in various fields. Fluorimetry relies on the phenomenon of fluorescence, where certain substances absorb light of a specific wavelength and then re-emit light at a longer wavelength. This process is critical as it lays the groundwork for quantitative analysis in various scientific disciplines. Moreover, the sensitivity and specificity of fluorimetry make it a preferred method in comparison to other spectroscopic techniques. Key aspects include the efficiency of energy transfer, the nature of the samples being analyzed, and the significance of the emitted light's properties.
Fluorescence: The Underlying Mechanism
Fluorescence occurs when a molecule absorbs photons of energy, leading to an excited state. After a brief, defined interval, the molecule releases energy as it returns to its ground state. This emission is typically observed as light of a longer wavelength, known as emission fluorescence. There are several factors influencing fluorescence intensity, including:
- Excitation wavelength: The light source used must match the absorption characteristics of the fluorophore.
- Environmental conditions: Factors such as temperature and solvent environment can affect the quantum yield, influencing the amount of light emitted.
- Molecular structure: Specific structural features of fluorophores determine their fluorescent properties. For instance, rigid structures often exhibit stronger fluorescence than their flexible counterparts.
Understanding these factors is essential for optimizing fluorimetric measurements for quantitative analyses in experiments.
Stokes Shift and Emission Spectra
The Stokes shift is a crucial concept in fluorimetry, referring to the difference in wavelength between the absorbed light and the emitted light. This shift occurs due to the transition from the ground state to the excited state and back again, with energy loss occurring in non-radiative processes during the excited state lifetime. The Stokes shift can often be advantageous, as it minimizes interference from the excitation light in measurement processes.
The emission spectrum is essential for assessing the performance of fluorimetric systems. It provides valuable details about the wavelengths of light emitted by a sample after excitation. The characteristics of the emission spectra give important insights into:
- Fluorophore identification: Different fluorophores exhibit unique emission spectra.
- Concentration determination: The intensity of the emitted light can be correlated to the concentration of the fluorophore in solution.
In summary, both the understanding of fluorescence mechanisms and the Stokes shift, along with the emission spectra, are indispensable for maximizing the potential of fluorimetry in scientific research.
Instrumentation in Fluorimetry Spectroscopy
Instrumentation plays a critical role in fluorimetry spectroscopy. It encompasses the various devices and systems necessary for conducting analyses based on fluorescence. Understanding this component is vital, as it affects the accuracy, sensitivity, and efficiency of measurements. Proper instrumentation is the backbone of successful fluorimetric analysis, allowing researchers to explore molecular interactions, identify substances, and quantify concentrations of analytes across diverse fields such as environmental science and biotechnology.
Components of Fluorimeters
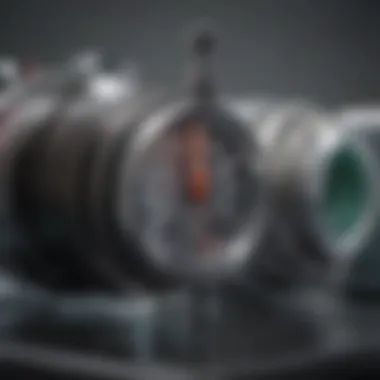
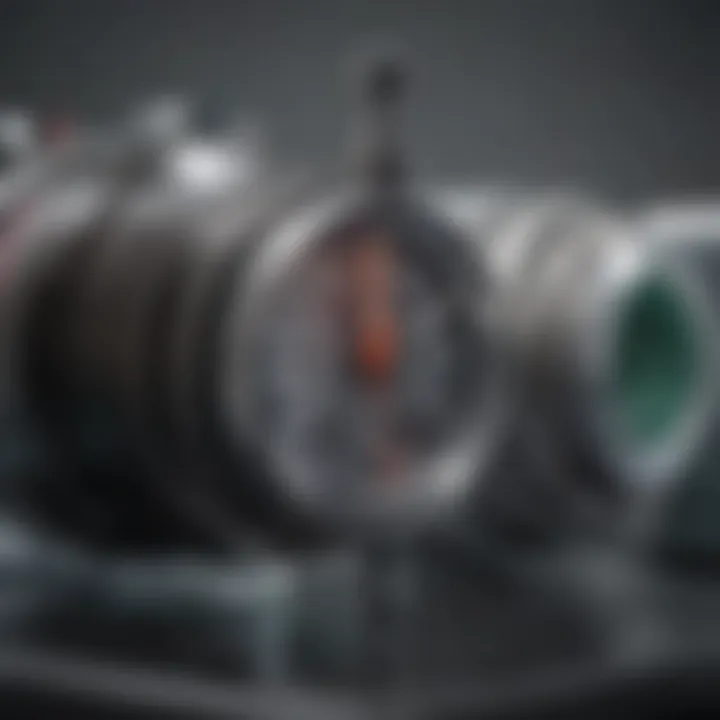
Fluorimeters are specialized instruments that detect the fluorescence emitted by samples. The main components include:
- Light Source: This is often a Xe lamp or a LED that provides the excitation light necessary for inducing fluorescence in the sample.
- Sample Holder: It securely holds the samples during analysis, ensuring stable positioning to reduce variations in readings.
- Filters: These optical components help in isolating the excitation wavelength from the emission wavelengths, improving measurement accuracy by minimizing signal interference.
- Monochromator or Spectrograph: These elements separate the emitted fluorescence into its constituent wavelengths for detailed analysis. They enhance the specificity of the measurements.
- Data Acquisition Systems: These convert the signals obtained from fluorescence into readable data for analysis.
Understanding each component's function enhances the capability to troubleshoot and optimize measurement processes in fluorimetry.
Detector Technologies
Detector technologies are crucial in fluorimetry as they convert the light signal emitted from the sample into electronic signals for analysis. Various detector types include:
- Photomultiplier Tubes (PMTs): Known for their high sensitivity, PMTs amplify weak signals and are widely used in traditional fluorimeters.
- Charge-Coupled Devices (CCDs): These detectors capture data in parallel, allowing for rapid measurements across multiple wavelengths, which is beneficial in complex analyses.
- Photodiodes: These are compact, reliable, and less expensive than PMTs, making them suitable for portable devices but with lower sensitivity.
- Avalanche Photodiodes (APDs): APDs provide high gain and are used in applications where time resolution is critical.
Choosing the right detector technology is essential for achieving optimal performance in fluorimetric experiments. The decision often depends on the specific analytical requirements, including sensitivity, speed, and cost considerations.
The instrumentation in fluorimetry directly influences the accuracy and reliability of spectroscopic measurements, making it indispensable in both research and practical applications.
The instrumentation in fluorimetry directly influences the accuracy and reliability of spectroscopic measurements, making it indispensable in both research and practical applications.
Sample Preparation Techniques
Sample preparation is a crucial step in fluorimetry spectroscopy. The quality of the results heavily depends on how samples are handled before analysis. In many instances, the inherent properties of the samples can interfere with the measurement of fluorescence. Hence, correct preparation techniques ensure that the samples provide reliable and reproducible data. The aim is to maximize the signal and minimize noise, while also considering the specificity of the techniques used.
Types of Samples Suitable for Analysis
Fluorimetry can analyze a wide variety of samples. Some common types include:
- Biological Samples: These can be cells, tissues, or bodily fluids. Fluorimetry can measure biomolecules like proteins or nucleic acids. These samples often require precise conditions.
- Environmental Samples: Water, soil, or air samples can be analyzed for pollutants. Fluorescent markers might be used to enhance sensitivity in detecting low concentrations of contaminants.
- Industrial Samples: In many manufacturing processes, staring materials and products can be evaluated for quality control. This ensures compliance with safety standards and product specifications.
When selecting samples, itβs essential to consider their fluorescence characteristics because some may exhibit background fluorescence, complicating the analysis.
Preparation Protocols
Preparation protocols vary significantly based on the sample type. Here are some general steps that are often incorporated:
- Sample Cleaning: Remove any contaminants that could interfere with the fluorescence measurement. For biological samples, this can include centrifugation to separate debris.
- Dilution: Adjust sample concentrations as needed. The goal is to attain a suitable concentration range for accurate measurement. For example, very concentrated samples can cause quenching, leading to misleading results.
- Buffering: Adding a buffer helps maintain pH stability during measurement. This is particularly critical for biological samples, as pH can greatly impact fluorescence.
- Incorporation of Fluorescent Dyes: In cases where samples have weak natural fluorescence, specific dyes or probes can be employed. These agents enhance sensitivity and specificity toward particular molecules of interest.
- Storage Conditions: Proper storage conditions, such as temperature and light exposure, must be maintained to avoid degradation. Samples should be stored at optimal conditions until analysis.
In summary, effective sample preparation plays a vital role in the successful application of fluorimetry spectroscopy. This section highlights the need for meticulous techniques to ensure accuracy and reliability in measurements.
Applications of Fluorimetry
The applications of fluorimetry spectroscopy are extensive and multifaceted. This analytical technique is not just limited to research environments; it has practical implications in various fields. Understanding how fluorimetry is applied enhances our grasp of scientific advancements in environmental science, healthcare, and industrial processes. It's important to note that the benefits of utilizing fluorimetry include high sensitivity, specificity, and fast response times. These factors significantly improve the effectiveness of analyses across different domains.
Environmental Monitoring
Environmental monitoring is one of the prominent use areas for fluorimetry. This technique allows researchers to detect and quantify pollutants in air, water, and soil samples with great accuracy. For example, fluorimetry can be used to measure concentrations of heavy metals, pesticides, and dyes. The fluorescent properties of many environmental contaminants permit their detection at very low concentrations, often in the nano or microgram per liter range.
Fluorescent indicators are particularly effective for this purpose. These indicators react with specific pollutants, yielding measurable fluorescent signals that correlate with the concentration of the pollutant. Besides assessing current levels of contamination, fluorimetry can also be used for monitoring changes over time, providing valuable data for trend analysis.
"Through careful monitoring and detection, fluorimetry plays a critical role in environmental protection and regulation."
"Through careful monitoring and detection, fluorimetry plays a critical role in environmental protection and regulation."
Biomedical Applications
In the biomedical field, fluorimetry has revolutionized diagnostics and research. This technique is vital for the detection of biomolecules, such as proteins and nucleic acids. Fluorimetry allows for the analysis of fluorescently labeled biological samples, enabling scientists to observe cellular functions and interactions at a molecular level.
Applications include fluorescence in situ hybridization (FISH), which visualizes specific nucleic acid sequences in tissues. Likewise, enzyme-linked immunosorbent assays (ELISAs) utilize fluorimetric techniques to quantify antigens or antibodies in samples. The sensitivity of fluorimetry is particularly valuable in early disease detection, therapeutic monitoring, and drug discovery processes. As a result, the integration of fluorescent probes in research optimizes the study of complex biological systems.
Industrial Uses
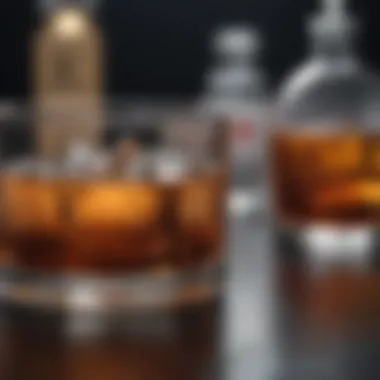
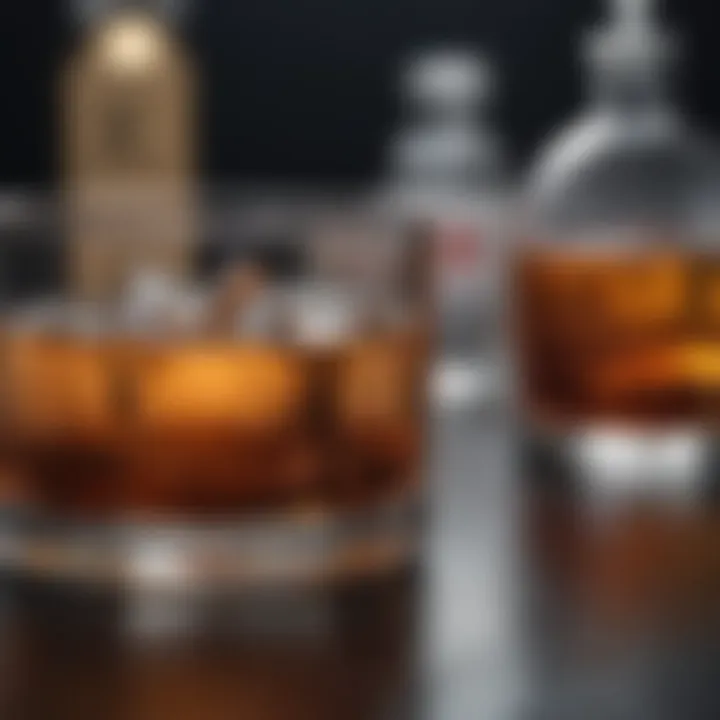
Industrial applications of fluorimetry demonstrate its versatility. This technique is employed for quality control and assurance in manufacturing processes. For instance, in the textile and dye industries, fluorimetry can measure residual dye in wastewater, ensuring compliance with environmental regulations. Additionally, the food and beverage sector utilizes fluorimetric techniques to detect specific contaminants or additives.
Beyond quality control, fluorimetry assists in material characterization. Polymers and other materials can be analyzed through their fluorescence properties, allowing for assessments of their composition and behavior under varying conditions. This capability offers critical insights for product development and optimization across multiple industries.
In summary, the applications of fluorimetry are expansive. From monitoring environmental safety and advancing biomedical science to ensuring industrial compliance and product quality, fluorimetry proves to be an indispensable tool. Its ability to provide quick and precise measurements remains a focal point for ongoing research and innovation.
Recent Innovations in the Field
Recent innovations in fluorimetry spectroscopy have significantly advanced its utility and application across various scientific domains. As the demand for more precise and efficient analytical methods increases, researchers have sought to enhance the capabilities of fluorimetric techniques. These innovations aim not only to improve measurement sensitivity and specificity but also to integrate fluorimetry with other analytical methods, creating versatile platforms for scientific inquiry.
Integration with Other Techniques
The integration of fluorimetry with other analytical techniques is a key development in recent years. By combining fluorimetry with methods such as chromatography or mass spectrometry, researchers can enhance the overall analytical capabilities. This multifaceted approach enables them to overcome some of the limitations typical of standalone fluorimetric methods. For instance, combining high-performance liquid chromatography (HPLC) with fluorimetric detection allows for the separation of complex mixtures before quantification. This integration enhances both detection limits and specificity, enabling the analysis of samples with overlapping fluorescent characteristics.
Moreover, the use of machine learning algorithms alongside fluorimetry is another innovation. These algorithms aid in interpreting the data, providing deeper insights into fluorescence patterns. Such integrations empower researchers to analyze large datasets efficiently, leading to more accurate and informed conclusions in various applications ranging from environmental monitoring to biomedical research.
Advancements in Instrumentation
The continuous advancement in instrumentation reflects another vital aspect of recent innovations in fluorimetry. Modern fluorimeters are now equipped with sophisticated detectors and optics, which significantly enhance sensitivity and accuracy. For example, the development of avalanche photodiodes and silicon photomultipliers provides higher quantum efficiency compared to traditional detectors. These improvements allow for the detection of lower concentrations of fluorescent species, making fluorimetry applicable in more challenging environments, such as trace-level detection in environmental samples.
Improvements in software for data acquisition and analysis also contribute to the evolution of fluorimetric techniques. Users now benefit from more intuitive interfaces and advanced algorithms that streamline the workflow, making it easier to perform complex analyses. Additionally, the advent of portable fluorimeters enables field testing, facilitating real-time data collection in situ, which is particularly valuable for environmental scientists and field researchers.
"As scientists continue to push the boundaries of fluorimetry, the integration of new detection technologies and data analysis methods will likely lead to even more groundbreaking applications across diverse fields."
"As scientists continue to push the boundaries of fluorimetry, the integration of new detection technologies and data analysis methods will likely lead to even more groundbreaking applications across diverse fields."
These advancements not only enhance the potential of fluorimetry but also set the stage for its increased adoption in practical applications. The dual focus on integration and instrumentation will continue to shape the future of fluorimetry spectroscopy, ensuring it remains a vital tool in scientific research.
Challenges and Limitations of Fluorimetry
Fluorimetry, like other analytical techniques, faces its own set of challenges and limitations that can affect the accuracy and reliability of results. Understanding these challenges is important for anyone involved in this field. By acknowledging the limitations, researchers and practitioners can take steps to mitigate their impact. This section evaluates key issues related to measurement interference, sensitivity, and specificity.
Interferences in Measurements
Interferences can arise from various sources and may compromise the integrity of fluorimetric analyses. Substances present in samples can emit fluorescence that overlaps with the target analyte's signal. This phenomenon can lead to incorrect quantification or misinterpretation of results. Examples include:
- Chemical Interferences: Compounds that fluoresce at similar wavelengths may obscure the signal of the intended target, complicating data interpretation. These substances could be contaminants or inherent properties of the matrix.
- Physical Interferences: Variations in sample temperature, pH, or solvent composition can alter the fluorescence characteristics, making results less reproducible.
To mitigate these issues, it is essential to understand the sample matrix and implement appropriate experimental controls. Techniques such as spectral scanning, where the emission spectrum is analyzed thoroughly, can help identify and reduce the impact of such interferences.
Sensitivity and Specificity Concerns
Sensitivity refers to the ability of a method to detect low concentrations of analytes, while specificity pertains to the ability to discern the desired probe from other substances present in the sample.
In fluorimetry, achieving high sensitivity while maintaining specificity can be particularly challenging. Some factors include:
- Fluorescence Quenching: This phenomenon, where the fluorescence intensity decreases due to the presence of quenchers, can lead to difficulties when measuring low concentrations. Understanding the sample's environment and making necessary adjustments is critical to overcoming this obstacle.
- Low Concentration Detection: For many applications, especially in biomedical research, the target analyte may exist in very low concentrations. Techniques employing highly tailored fluorescent tags or probes can enhance sensitivity but may not always guarantee specificity.
Maintaining a balance between sensitivity and specificity remains a core challenge in the field. Continuous improvement in detector technologies and fluorophore design is taking place. For a robust analysis, both aspects must be considered and addressed accordingly.
"Challenges in fluorimetry, if navigated carefully, do not serve as roadblocks but as avenues for innovation and improvement in analytical science."
"Challenges in fluorimetry, if navigated carefully, do not serve as roadblocks but as avenues for innovation and improvement in analytical science."
Future Directions in Fluorimetry Research
The field of fluorimetry spectroscopy is continuously evolving. Understanding future directions in this area is crucial for advancing scientific knowledge and applications. Researchers and practitioners must stay informed about what lies ahead. This section will address emerging trends, technologies, and potential new applications, highlighting their significance in the broader context of fluorimetry research.
Emerging Trends and Technologies
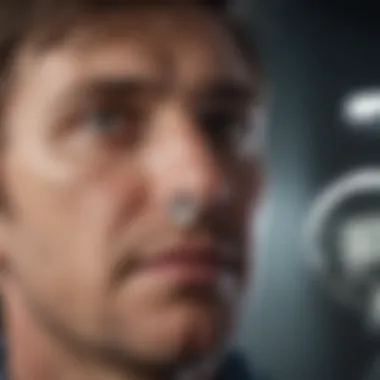
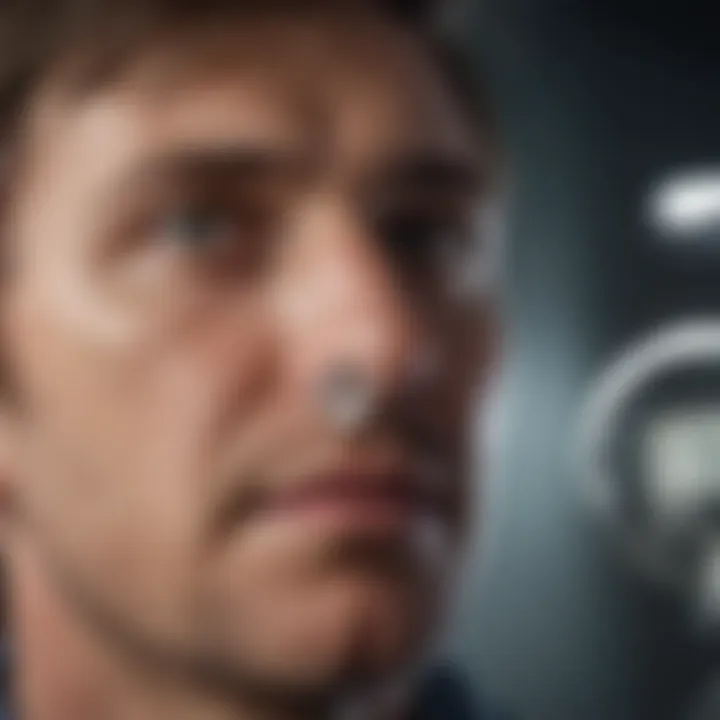
Recent advancements in technology have opened new avenues for fluorimetry applications. Among these developments, microfluidics is gaining traction. Microfluidics enables the manipulation of small volumes of fluids, improving the efficiency and sensitivity of fluorimetric measurements.
Another trend is the integration of machine learning with fluorimetry data analysis. This approach allows for better pattern recognition in complex samples. It can enhance data interpretation and improve the accuracy of results.
Nanotechnology is also making a significant impact. The use of nanomaterials can increase the sensitivity and selectivity of fluorimetric approaches. Quantum dots, for instance, are becoming prominent due to their unique optical properties. They offer potential improvements in detection limits and spectral range.
Moreover, portable fluorimeters are being developed for field applications. These devices provide quick, on-site analyses, making fluorimetry accessible in various environments. Researchers can conduct studies in real-time, leading to faster decision-making processes.
Potential New Applications
The versatility of fluorimetry allows for innovative applications across many fields. One promising area is in health monitoring. Fluorimetric methods can be applied to detect biomarkers for diseases. This capability can help in early diagnosis and personalized medicine.
Environmental monitoring is another vital application. Fluorimetry can be used to track pollutants in water sources. Real-time monitoring can provide valuable data for policymakers and researchers to manage environmental health.
In the field of food safety, fluorimetric techniques can identify contaminants. This application ensures the safety and quality of food products. It can lead to better regulation and consumer protection.
Lastly, the integration of fluorimetry with genomic studies is a developing area. It has the potential to impact fields such as biotechnology. By illuminating and quantifying nucleic acid interactions, researchers can gain insights into genetic mechanisms.
"The future of fluorimetry spectroscopy relies on innovation. Emerging technologies and potentiaI applications can revolutionize scientific fields."
"The future of fluorimetry spectroscopy relies on innovation. Emerging technologies and potentiaI applications can revolutionize scientific fields."
Overall, these future directions hold promise for enhancing the practical utility of fluorimetry spectroscopy. They represent a dynamic and promising frontier, vital for harnessing the full potential of this analytical technique.
Comparative Analysis with Other Spectroscopic Techniques
Understanding spectroscopic techniques is essential for students, researchers, and professionals. Each method has its unique strengths and weaknesses, which determine its suitability for specific applications. A comparative analysis allows one to appreciate the different approaches, helping to select the correct method for the job at hand. This section focuses on comparing fluorimetry to UV-Vis and Raman spectroscopy, two methods frequently used in chemical and biological analysis.
UV-Vis Spectroscopy
UV-Vis spectroscopy, or ultraviolet-visible spectroscopy, measures the absorption of light in the ultraviolet and visible regions of the electromagnetic spectrum. The primary use of this technique is to determine the concentration of a solute in a sample. It relies on the principle that different molecules absorb light at specific wavelengths.
One critical advantage of UV-Vis spectroscopy is its speed and simplicity. This technique often requires minimal sample preparation compared to fluorimetry, making it accessible for routine analyses. Several compounds, particularly those with conjugated double bonds, show significant absorption, which can be quantified easily.
However, UV-Vis spectroscopy does have limitations. It is not as sensitive as fluorimetry. When analyzing low-concentration samples, the results may be less reliable. Moreover, the range of detectable compounds is narrower with UV-Vis; some materials do not exhibit strong absorbance in the UV-Vis range, which could limit the analysis.
The effectiveness of UV-Vis spectroscopy is evident in applications across various fields, including environmental monitoring and pharmaceutical studies, where rapid and straightforward measurements are critical.
The effectiveness of UV-Vis spectroscopy is evident in applications across various fields, including environmental monitoring and pharmaceutical studies, where rapid and straightforward measurements are critical.
Raman Spectroscopy
Raman spectroscopy relies on inelastic scattering of monochromatic light, typically from a laser. This technique provides molecular information, giving insights into the vibrational modes of molecules. It is particularly useful in characterizing organic and inorganic materials.
One substantial advantage of Raman spectroscopy is that it can provide both qualitative and quantitative chemical information without needing extensive sample preparation. Unlike fluorimetry, which often requires fluorescent markers, Raman can analyze samples in their natural state. This property makes it valuable in applications such as material science and forensic analysis.
However, Raman may face challenges regarding sensitivity. Fluorescence can frequently mask Raman signals when analyzing certain biological samples, complicating interpretations. Furthermore, the high cost of Raman instrumentation compared to fluorimeters can be a barrier for labs with limited budgets.
Ending
In this article, we explored the intricate realm of fluorimetry spectroscopy. It is essential to understand the core aspects of this technique for various reasons. First, it has significant implications in multiple fields, including environmental science, biochemistry, and material sciences. The ability to measure fluorescent emissions enables researchers to gain insights into molecular behaviors and interactions. Through comprehensive knowledge of instrumentations, sample preparation techniques, and applications, one can appreciate the utility of fluorimetry in both research and industry.
Moreover, the challenges and limitations outlined in the discussions shed light on areas requiring further attention. Addressing measurement interferences and improving sensitivity can enhance the reliability of results. Thus, it is critical to take these findings and integrate them into further research efforts.
Fluorimetry is not just a technique; it is a lens through which complex interactions can be analyzed and understood.
Fluorimetry is not just a technique; it is a lens through which complex interactions can be analyzed and understood.
Summary of Findings
This article highlights the fundamental principles that govern fluorimetry spectroscopy, emphasizing the importance of understanding fluorescence and the Stokes shift. The instrumentation section provided insights into the key components of fluorimeters and the technologies used for detection. Different application domains were covered, showcasing the versatility of fluorimetry and its significant impact on environmental monitoring, biomedical applications, and industrial uses. The recent advancements discussed highlight how innovation continues to drive the field forward.
Implications for Future Research
Looking ahead, the implications for future research in fluorimetry are vast. One promising area is the integration of fluorimetry with other analytical techniques. This convergence can lead to more comprehensive assessment methods, as seen in its combination with UV-Vis spectroscopy and mass spectrometry. Additionally, researchers should focus on developing more sensitive and specific detection methods to tackle current limitations. New applications, such as real-time monitoring of biological systems or pollutant tracking in environmental studies, also hold great potential for exploration. As researchers continue to push boundaries in technology and applications, fluorimetry will undoubtedly remain a vital tool in scientific investigation.