Understanding H2S Oxidation: Mechanisms and Implications
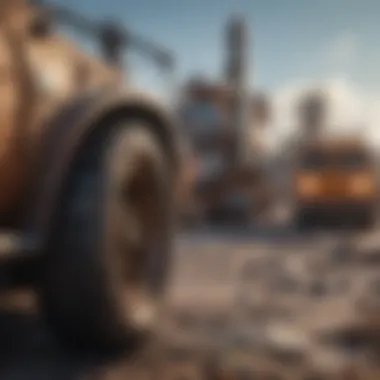
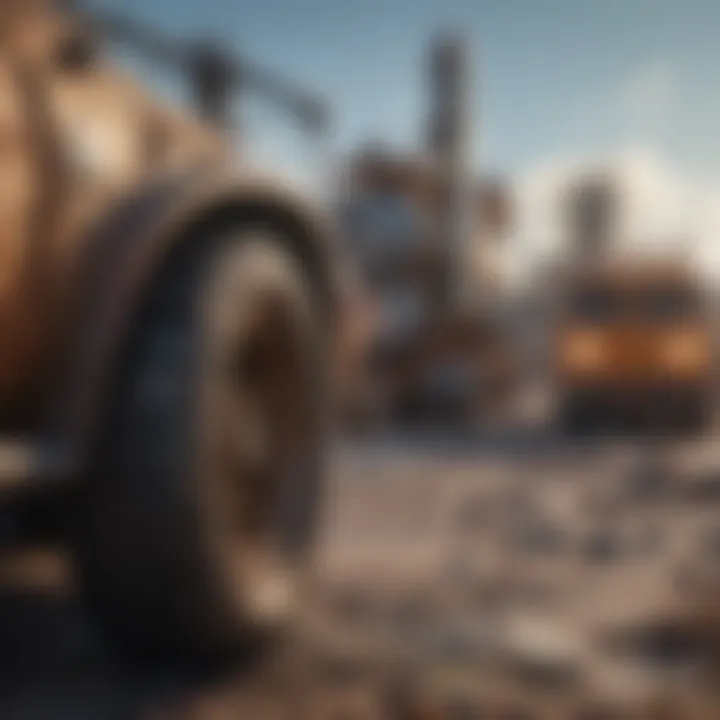
Intro
In a world where chemical interactions define ecological balance, understanding hydrogen sulfide (S) oxidation becomes crucial. S, often recognized for its characteristic foul odor reminiscent of rotten eggs, is a compound of significant interest, both in the realm of natural sciences and industrial applications. Delving into its oxidation process reveals not only intriguing biochemical pathways but also the broader environmental and health implications.
S often originates from various natural processes, including the decomposition of organic matter and volcanic activity. Yet, human activities such as waste management and fossil fuel extraction also contribute to its presence. The oxidation of S is a complex process, blending biochemistry with environmental science. This article aims to peel back the layers of this complex chemical story, exploring the mechanisms involved and the broader implications—environmental balance, health risks, and innovative biotechnological applications.
By unpacking key concepts, current research, and practical implications, readers will gain a deeper appreciation of S oxidation and its role in sustainability and industry.
Intro to S Oxidation
Hydrogen sulfide (S) oxidation is a crucial biochemical process that has garnered increased attention due to its implications across different scientific fields, from environmental science to human health. It serves as a bridge connecting various biochemical pathways and ecological dynamics, enabling a detailed understanding of how sulfur behaves in natural systems and industrial applications. This process not only plays a vital role in the sulfur cycle, but also influences global carbon cycling and can affect atmospheric chemistry.
Defining Hydrogen Sulfide
S is a colorless gas known for its distinct and unpleasant odor, reminiscent of rotten eggs. Commonly found in natural gas, biogas, and as a byproduct of various industrial processes, such as petroleum refining or wastewater treatment, hydrogen sulfide presents both environmental challenges and opportunities. Its concentration in natural habitats can signal geological or biochemical conditions; however, at elevated levels, S becomes toxic and poses risks to both human health and ecosystems.
S is classified as a weak acid, reacting with strong bases to form sulfide salts. Despite its noxious nature, S is produced naturally through the decay of organic matter, offering essential nutrients to certain microorganisms. Understanding its definitive traits helps us grasp the significance of its oxidation.
Importance of Oxidation in Biological Systems
The importance of oxidation in biological systems cannot be overstated. It is through oxidation that S is converted into less harmful substances, playing a critical role in various metabolic pathways. This process is essential for life; not only does it help detoxify S under certain conditions, but it also enables organisms to harness energy from sulfur compounds.
Oxidation enhances the viability of natural environments, as it aids in recycling nutrients. For instance, sulfur-oxidizing bacteria utilize S as an energy source, converting it into elemental sulfur or sulfate, which are crucial for primary productivity in ecosystems.
Moreover, the dynamic between S oxidation and oxygen availability can shape entire habitats. In oxygen-replete zones, S is oxidized effectively, fostering rich biodiversity. In contrast, in hypoxic environments, S accumulation poses risks that challenge the stability of these ecosystems.
In essence, hydrogen sulfide oxidation is not just a process; it is a life-sustaining mechanism that interweaves with the very fabric of ecological integrity.
In essence, hydrogen sulfide oxidation is not just a process; it is a life-sustaining mechanism that interweaves with the very fabric of ecological integrity.
In summary, grasping the fundamentals of S oxidation sets the stage for understanding broader biochemical pathways, microbial interactions, and environmental implications, all of which will be further explored in this article.
Biochemical Mechanisms of S Oxidation
The biochemical mechanisms of hydrogen sulfide (S) oxidation are at the core of understanding how this compound interacts with various biological and environmental systems. Recognizing these mechanisms not only illuminates the process itself but also highlights how S plays a dual role – as both a harmful pollutant and a potential benefactor in certain ecosystems. This section aims to shed light on the enzymatic pathways and the redox reactions that govern S oxidation, providing insights into their significance in shaping ecological dynamics and human health.
Enzymatic Pathways Involved
Sulfide-Quinone Oxidoreductase
Sulfide-quinone oxidoreductase is a pivotal enzyme in the oxidative metabolism of S. This enzyme facilitates the transfer of electrons during the oxidation process, leading to the formation of sulfur compounds that are less toxic and more manageable for biological systems. One of the key characteristics of sulfide-quinone oxidoreductase is its efficiency in catalyzing reactions even at low concentrations of S. This makes it invaluable when assessing the microbial oxidation of sulfide in various environments, such as marine ecosystems.
A unique feature of this enzyme is its versatility, as it can operate across different redox states, adapting to varying environmental conditions. Its beneficial aspects lie in its ability to function in both prokaryotic and eukaryotic organisms, thus underscoring its evolutionary significance. However, there are some disadvantages; its activity can be hindered by toxic compounds, leading to potential bottleneck effects in S oxidation pathways under certain conditions.
Thiosulfate Sulfurtransferase
Thiosulfate sulfurtransferase plays an equally crucial role in S oxidation pathways. This enzyme is primarily responsible for reducing thiosulfate to sulfite while simultaneously transferring sulfur atoms to other acceptors. The key characteristic of thiosulfate sulfurtransferase is its dual functionality – it not only participates in S oxidation but also in sulfur metabolism, thus linking various elemental cycles closely.
The unique feature of this enzyme is its broad substrate specificity, which allows it to interact with several sulfur compounds, enhancing its utility in various biochemical contexts. In terms of advantages, its efficiency in catalyzing reactions has made it a focal point in studies aimed at understanding sulfur management in the environment. However, a notable drawback can be that high concentrations of oxidized sulfur compounds may inhibit its function, which can lead to disrupted sulfur cycling in specific ecosystems.
Redox Reactions in S Oxidation
The role of redox reactions in S oxidation cannot be overstated. These reactions are fundamental in transforming S into sulfur or sulfate, critical components in various biochemical and ecological processes. Redox reactions involve the transfer of electrons, where S acts as a reductant, donating electrons, while other substances, often oxygen or metal ions, serve as oxidants.
Through these reactions, S is converted first into intermediate compounds such as thiosulfate, then further into sulfate, completing the oxidation process. The efficiency of these reactions is influenced by the availability of electron acceptors, pH levels, and the presence of specific microorganisms that can catalyze the necessary biochemical transformations. Understanding these redox couples is essential not only for grasping the fundamental principles of S oxidation but also for developing potential biotechnological applications aimed at mitigating the environmental impacts of S.
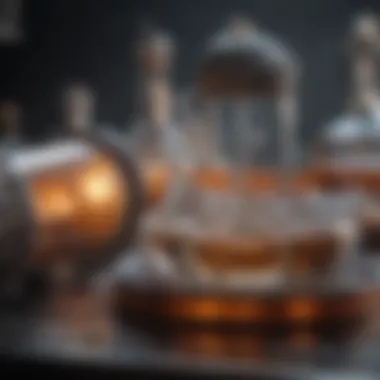
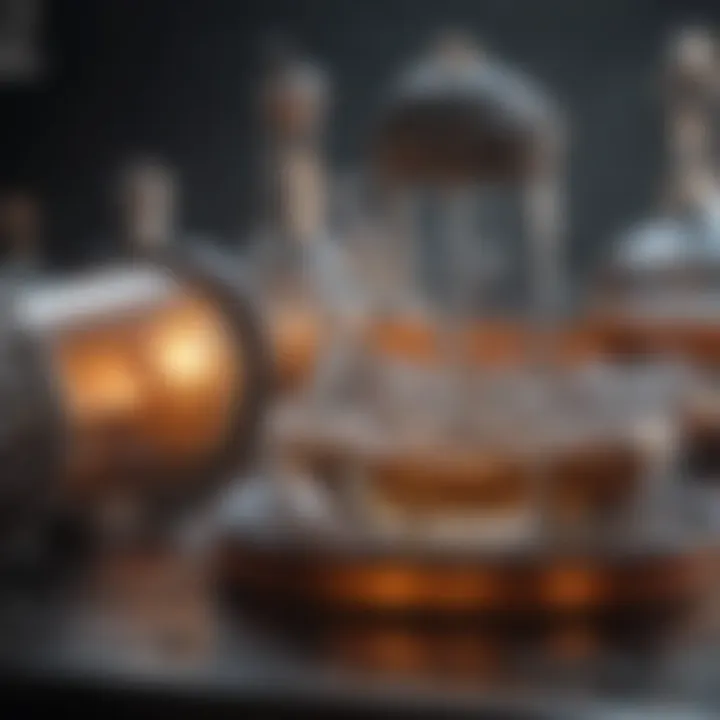
Microbial Roles in S Oxidation
Hydrogen sulfide oxidation is not just a chemical process; it’s a vibrant interplay of life at the microbial level. Microorganisms play a critical role in the cycling of S, transforming it into different sulfur compounds that benefit the ecosystem. This topic is essential for understanding not only the biochemical pathways involved in S oxidation but also the larger implications for nutrient cycling in various environments, from oceans to wetlands. The microbes that take center stage in this narrative help maintain ecological balance, and their interactions can significantly influence environmental health.
Key Microorganisms Involved
Chemolithotrophic Bacteria
Chemolithotrophic bacteria are fascinating players in the S oxidation game. These microorganisms have the unique ability to derive energy from inorganic compounds, which makes them specially suited for environments where organic matter may be scarce. One key characteristic of chemolithotrophic bacteria is their capacity to oxidize hydrogen sulfide, using it as an electron donor in energy production.
They’re a beneficial choice for discussions around microbial roles in S oxidation because they actively participate in various biogeochemical cycles. A unique feature of these bacteria is their energy-efficient metabolic pathways, which can sustain high rates of S oxidation. However, one must note that while these processes are efficient, they typically require specific environmental conditions, such as alkaline pH levels and low temperatures, which can limit their distribution.
Sulfur-Oxidizing Bacteria
Sulfur-oxidizing bacteria (SOB) are another essential group involved in S oxidation. They thrive in sulfur-rich environments and are known for their distinct capability to convert sulfur compounds into sulfate. This physiological property not only aids in detoxifying environments rich in hydrogen sulfide but also contributes to the overall sulfur cycle in ecosystems. One of the reasons they are a popular choice for this article is their ecological significance in bioremediation efforts and wastewater treatment.
A unique feature of sulfur-oxidizing bacteria is their versatility in habitat; they can be found in various environments, from deep-sea hydrothermal vents to agricultural soils. However, while they play a pillar role in enhancing soil fertility by converting sulfide into sulfate, high concentrations of S can be toxic to them, thus presenting an environmental challenge.
Symbiotic Relationships
The relationships that some microorganisms form with hosts, including plants and animals, further enhance the complexity of S oxidation processes. These symbiotic interactions offer more than just benefits to the microbes; they often supply essential nutrients to their hosts, creating a reciprocal relationship that maximizes the overall efficiency of nutrient cycling. In understanding S oxidation, it is vital to consider how these relationships can influence the microbial community structure and function in different environments.
Environmental Impacts of S Oxidation
The environmental consequences of hydrogen sulfide (S) oxidation are substantial, impacting ecological systems and biogeochemical interactions. S oxidation plays a pivotal role in maintaining the balance of sulfur compounds in the environment. Understanding these impacts helps grasp how this process influences our ecosystems and the broader implications for environmental health.
Role in Biogeochemical Cycles
Sulfur Cycle
The sulfur cycle is an intricate network of processes that revolve around the conservation and transformation of sulfur in various forms. At its core, the oxidation of S is essential in converting this toxic gas into sulfate, which is more stable and less harmful. This transformation is fundamental to the cycle, as it bridges the gap between the anaerobic degradation of organic matter and the aerobic processes involving sulfur oxidizers.
One of the key characteristics of the sulfur cycle is its closed-loop nature, allowing sulfur to be recycled continuously. This feature is particularly beneficial as it ensures the availability of sulfur, an essential nutrient for many organisms in the environment.
A distinct advantage of understanding the sulfur cycle in the context of S oxidation is its implications for soil health. The oxidation processes enrich soil sulfate levels, contributing to agricultural productivity. However, these interactions can also lead to disadvantages, such as the potential for acidification if the balance tips excessively towards oxidation.
In summary, the sulfur cycle's role in transforming S into non-toxic sulfate showcases its significance in ecological stability yet urges caution regarding over-oxidation.
Carbon Cycle
Similarly, the carbon cycle is intertwined with S oxidation, playing a crucial part in the interrelationship between carbon and sulfur in the environment. S oxidation can indirectly influence carbon cycling by affecting how organic matter decomposes and how carbon is stored in various ecological systems.
What stands out about the carbon cycle is its dynamic and synergistic nature with the sulfur cycle. The oxidation of S can lead to increased availability of carbon-based substrates for microorganisms, thus enhancing CO2 release through respiration. This creates a balance where carbon and sulfur are constantly exchanged.
A unique feature of this interconnectedness lies in its broader implications for climate change. While S oxidation can enhance certain carbon cycling processes, it may also contribute to methane emissions if anaerobic conditions are favored during sulfur oxidation. This relationship can create challenges for researchers trying to mitigate greenhouse gas emissions.
To summarize, the carbon cycle's intricate relationship with S oxidation highlights both opportunities for enhanced nutrient cycling and challenges regarding greenhouse gas emissions.
Effects on Ecosystems
S oxidation has profound effects on ecosystems, influencing everything from microbial dynamics to nutrient availability. The presence of S in environments can lead to toxic conditions, which, when oxidized, shifts the dynamics towards healthier system functionality.
- Microbial Diversity: The microbial communities involved in S oxidation often exhibit high levels of diversity, which enhances ecosystem resilience.
- Nutrient Availability: Well-balanced S oxidation ensures that nutrients remain accessible for plant growth and supports the resilience of food webs.
Ultimately, the environmental impacts of S oxidation are complex, signifying the need for ongoing research to understand and manage these biochemical processes effectively. Both the sulfur and carbon cycles reflect the ongoing dance of nature, with S oxidation acting as a vital player in ensuring balance and health within ecosystems.
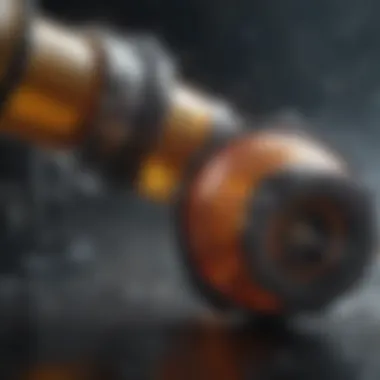
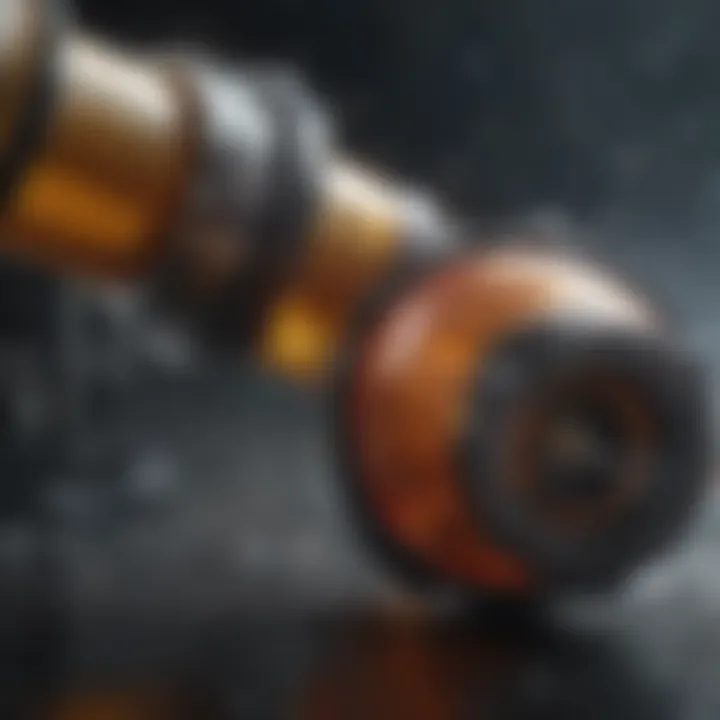
"Understanding how S oxidation affects biogeochemical cycles provides insight not only into environmental dynamics but also into potential agricultural practices and climate change mitigation strategies."
"Understanding how S oxidation affects biogeochemical cycles provides insight not only into environmental dynamics but also into potential agricultural practices and climate change mitigation strategies."
For further insights on the sulfur cycle and its implications, visit Wikipedia's page on the Sulfur Cycle or read about its environmental engagements on Britannica.
Human Health Considerations
In discussions surrounding hydrogen sulfide (S) oxidation, the implications for human health emerge as a critical area of focus. This section delves into the dual nature of S: on one hand, it’s recognized for its toxicity, while on the other, it presents potential therapeutic avenues. Understanding these facets is essential for students, researchers, educators, and professionals engaged in health sciences and environmental studies.
Toxicity of S and Its Oxidation Products
Human exposure to hydrogen sulfide is a cause for considerable concern. S is notorious for its characteristic odor, often described as rotten eggs, but its dangers far exceed olfactory offenses. When inhaled at high concentrations, it can lead to:
- Immediate health effects: Symptoms like irritation of the eyes, throat, and respiratory system can manifest almost instantly.
- Neurological impacts: Prolonged exposure can result in headaches, dizziness, and in severe cases, loss of consciousness.
The toxicity isn’t limited merely to the gas itself but extends to its oxidation products as well. For example, sulfur dioxide (SO2), a byproduct of the oxidation process, can cause lung and cardiovascular issues. Each oxidative transformation may yield compounds that interact harmfully with human cellular mechanisms, making monitoring and managing exposure a necessity in various industrial and ecological settings.
"The effects of hydrogen sulfide are dependent on concentration and duration of exposure. Even at low levels, it's a subtle villain; its presence can create a toxic environment without immediate warning."
"The effects of hydrogen sulfide are dependent on concentration and duration of exposure. Even at low levels, it's a subtle villain; its presence can create a toxic environment without immediate warning."
Potential Therapeutic Applications
Contrasting its hazardous nature, S and its oxidized products have also garnered attention for their potential therapeutic applications. Some significant aspects include:
- Vascular effects: Research indicates that S can play a role in vasodilation, providing possible treatments for conditions like hypertension.
- Neuroprotection: There’s evidence suggesting S might protect against neurodegenerative diseases by modulating oxidative stress.
Scientists are exploring these therapeutic avenues more rigorously. For example:
- S Pharmaceuticals: Dexamethasone, known for its anti-inflammatory properties, can show synergy when paired with S. This demonstrates a pathway for developing treatments for respiratory diseases.
- Clinical Trials: Studies are underway to evaluate the effectiveness of S donors in various medical conditions, revealing how this gas can shift from villain to ally when properly harnessed.
As researchers continue to deepen their understanding, it becomes clear that S isn't just a toxin but also holds significant promise in the realm of medicine. Notably, the balance between its risks and benefits underscores the necessity for comprehensive research in both environmental and health contexts.
Analytical Techniques for S Oxidation
Understanding hydrogen sulfide oxidation goes beyond just its biochemical mechanisms; it involves a variety of analytical techniques crucial for measuring and interpreting these reactions in both laboratory and environmental settings. These methods can provide insight into the extent of oxidation, the intermediate compounds formed, and their subsequent fate in various systems. Therefore, selecting appropriate analytical techniques is paramount since it influences the accuracy of data collection and interpretation, directly impacting our understanding of S dynamics.
Measurement Methods
Gas Chromatography
Gas chromatography is a widely used technique in the analysis of S and its oxidation products. This method allows for the separation and quantification of volatile compounds, providing a thorough analysis of gas samples collected from various environments. One key characteristic of gas chromatography is its sensitivity; it's able to detect concentrations of S in parts per million, making it a go-to choice for researchers studying air quality and emissions.
A unique feature of gas chromatography is its ability to efficiently separate mixtures. This separation enables researchers to analyze multiple sulfur compounds in a single run, offering a comprehensive overview of S's behavior during oxidation. However, while it brings distinct advantages, it also has some drawbacks. For instance, the equipment can be costly and requires skilled personnel to operate effectively.
Electrochemical Sensors
Electrochemical sensors present another powerful option for analyzing S oxidation. These devices typically measure the current that results from the oxidation of the hydrogen sulfide, providing real-time data. One key characteristic of electrochemical sensors is their portability, which allows them to be deployed in the field rather than confined to a lab setting. This is particularly beneficial for environmental monitoring and ensures immediate data capture during experiments.
The unique feature of electrochemical sensors lies in their rapid response time; they can quickly detect changes in S concentrations, offering crucial insights into dynamic systems. Nevertheless, the reliance on these sensors raises some considerations. They can be affected by other gases in the environment, potentially leading to inaccurate readings. It's therefore essential to calibrate them correctly and understand their limitations when interpreting data.
Challenges in Analysis
Analyzing S oxidation presents several challenges that can complicate results. Variability in sample composition, interference from other compounds, and the instability of S in certain conditions can skew results. Additionally, because S is toxic and can pose safety risks, working with it requires strict safety protocols, which may limit the practicality of some analytical methods.
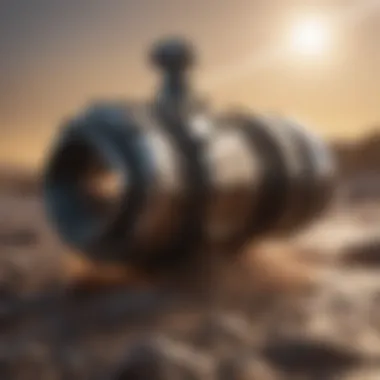
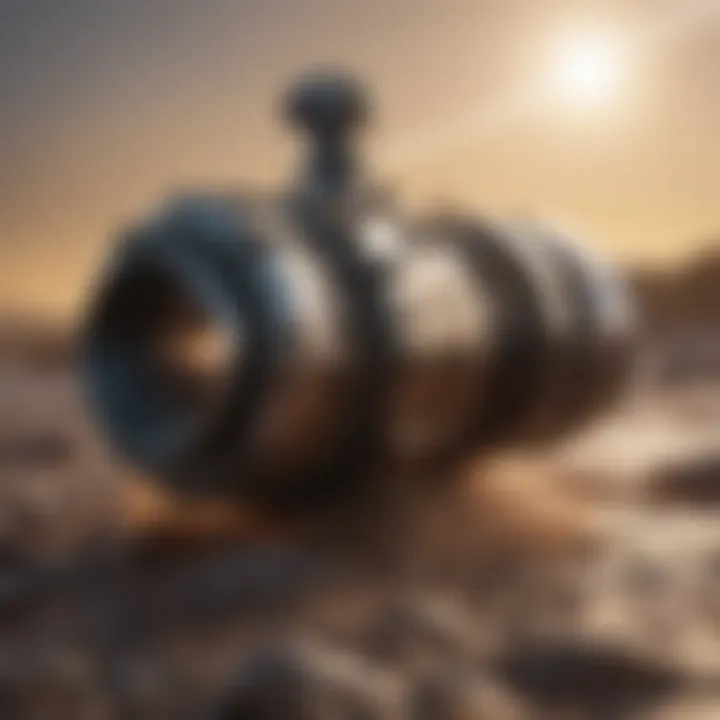
The analytical landscape for S is not without its hurdles, but advancing technologies continue to address these challenges, improving the quality and reliability of results. As techniques evolve, our ability to assess hydrogen sulfide oxidation will undoubtedly enhance, offering deeper insights into its implications across biological and environmental contexts.
Technological Applications of S Oxidation
The oxidation of hydrogen sulfide (S) presents significant technological opportunities across various industries. Understanding and harnessing the oxidation pathways of S can lead to advancements in environmental management, waste treatment, and even energy production. The relevance of this topic spans numerous applications, each of which contributes to sustainability and efficiency in technological processes.
Bioremediation Strategies
Bioremediation employs living organisms to mitigate or eliminate pollutants from the environment, and S oxidation plays a pivotal role in this context. Certain microorganisms, particularly sulfur-oxidizing bacteria, are effective in transforming S into less harmful compounds, thus addressing pollution issues tied to sulfur and its derivatives.
Implementing bioremediation strategies involves several steps:
- Microbial Selection: Identifying specific strains such as Thiobacillus and Beggiatoa that thrive in S-rich environments is crucial. These bacteria utilize S as an energy source, oxidizing it to sulfate or sulfur for their growth.
- Environmental Conditions: Optimizing conditions like pH, temperature, and nutrient levels enhances the efficiency of microbial activity. Conditions must be tailored to favor the selected microbes, ensuring a higher degradation rate of S.
- Application Methods: There are various approaches to introduce these microorganisms into contaminated sites. Techniques like bioaugmentation or biostimulation can be employed. In bioaugmentation, specific microorganisms are added, whereas biostimulation involves providing nutrients to boost native microbial populations.
"The success of bioremediation hinges not only on microbial capabilities but also on the understanding of the local ecosystem’s dynamics."
"The success of bioremediation hinges not only on microbial capabilities but also on the understanding of the local ecosystem’s dynamics."
These strategies offer environmentally friendly solutions, helping to restore polluted sites while minimizing harm to the ecosystem.
Industrial Applications
Industrial processes utilizing S oxidation have gained traction, especially in sectors like energy and materials production. Here are some noteworthy applications:
- Wastewater Treatment: S is often a byproduct in various industrial processes. Oxidation helps in decontaminating wastewater, reducing sulfur compound levels, thus preventing foul odors and toxicity that can upset aquatic life.
- Sulfur Recovery: In refineries and natural gas processing plants, S can be converted to elemental sulfur through oxidation processes. This not only provides a resource—sulfur—but also curtails environmental pollution resulting from S emissions.
- Energy Generation: Harnessing the resulting energy from S oxidation in biological systems can be transformative. Emerging technologies focus on using sulfur-oxidizing bacteria in bioenergy systems that produce electricity as they oxidize S, leading to sustainable energy generation.
- Production of Sulfate Compounds: Various industries rely on sulfate chemicals, which are essential in manufacturing fertilizers and detergents. Oxidizing S to sulfate fosters a lighter environmental footprint for production processes.
The applications of S oxidation in industrial settings illustrate pathways toward sustainability and improved efficiency across sectors. Understanding these technologies is vital for future advancements and responsible resource management, allowing industries to progress while reducing their environmental impact.
Research Advances in S Oxidation
The landscape of S oxidation research is broad and dynamic, with significant implications for both environmental and health sectors. As awareness increases regarding the crucial functions hydrogen sulfide plays in biological systems and its potential hazards, the drive for understanding its oxidation mechanisms has intensified. Recent findings demonstrate not just the biochemical pathways involved but also the environmental significance of these processes, thus making this area ripe for exploration.
Recent Discoveries
Several recent breakthroughs have expanded our understanding of S oxidation. Research has unveiled new enzymatic pathways that were previously misunderstood or overlooked. Notably, scientists have identified previously unrecognized sulfur-oxidizing bacteria capable of catalyzing S conversion in extreme environments, such as hydrothermal vents and anoxic sediments. These microorganisms, like Arcobacter and Beggiatoa, are proving essential not only for biogeochemical cycles but also for biotechnological applications in nutrient cycling and wastewater treatment.
Furthermore, innovations in biochemical analysis have allowed researchers to map the interactions between S oxidation and various metabolic processes. For example, recent studies have explored the role of thiosulfate in S detoxification, revealing how certain pathways can be harnessed to develop new bioremediation techniques. This understanding can lead to better methods for reclaiming contaminated environments, further underscoring the value of ongoing research in this field.
"Understanding microbial mechanics in S oxidation serves as a cornerstone for ecological restoration and health advancement."
"Understanding microbial mechanics in S oxidation serves as a cornerstone for ecological restoration and health advancement."
Future Directions in Research
Looking ahead, the prospects for S oxidation research seem promising. There are several areas that require further investigation:
- Genetic Engineering: Advances in synthetic biology may allow for the creation of engineered microorganisms with optimized pathways for S oxidation. Such innovations could enhance bioremediation efforts in areas heavily affected by sulfur compound pollution.
- Metagenomic Approaches: Using metagenomics to better understand diverse microbial communities involved in S oxidation can provide insights into how these microorganisms interact ecologically and metabolically.
- Climate Change Implications: With global changes in climate, exploring the responses of S oxidizing processes under varying environmental conditions holds great importance. This could provide critical data relevant to sulfur cycling and its subsequent effects on climate.
- Health Implications: Understanding the outcomes of S oxidation on human health, particularly concerning its therapeutic applications, poses a significant research direction. Investigating how oxidative stress from S affects cellular systems could lead to novel medical treatments or preventive measures against toxicity.
As we continue to peel back the layers of this multifaceted process, researchers will play an essential role in understanding not just how S is oxidized, but also its broader implications and applications in multiple fields.
Finale
In contemplating the vast landscape of S oxidation, it becomes evident that this process serves as a cornerstone for a myriad of ecological and technological advancements. The significance of understanding hydrogen sulfide oxidation lies not only in its biological implications but also in its role as a bridge connecting various scientific disciplines. From the mechanisms underpinning enzymatic pathways to the environmental ramifications, each facet plays into broader narratives of sustainability and health.
Summary of Key Points
The exploration of S oxidation has unveiled several key insights:
- Biochemical Pathways: Understanding the enzymatic pathways such as sulfide-quinone oxidoreductase and thiosulfate sulfurtransferase enhances our grasp of how sulfide ions are transformed and utilized.
- Microbial Contributions: The pivotal role that specific microorganisms play in S oxidation highlights the interconnectedness of life forms, where chemolithotrophic and sulfur-oxidizing bacteria are essential players in sulfur cycles.
- Environmental Relevance: The implications for global biogeochemical cycles, such as the sulfur and carbon cycles, showcase S oxidation's role in maintaining ecological balance, particularly in anaerobic environments.
- Human Health: Understanding both the toxicity and potential therapeutic applications of S and its products opens new avenues for medical research and environmental health strategies.
- Technological Applications: From bioremediation strategies to industrial uses, leveraging S oxidation can lead to innovative biotechnological solutions that address industrial pollution and waste management issues.
Final Thoughts on S Oxidation
As we wrap our heads around the complexities of S oxidation, it’s clear that this topic extends beyond the laboratory or classroom walls. The interactions, pathways, and consequences of S oxidation entwine with ecological dynamics as well as human innovation. A thoughtful approach to studying S oxidation not only enriches our scientific knowledge but encourages us to consider new possibilities for technological applications. By diving deep into these biochemical mechanisms and recognizing their implications, we stand on the precipice of breakthroughs that can influence both environmental stewardship and human health.