Exploring the Depths of Optical Metallurgical Microscopes
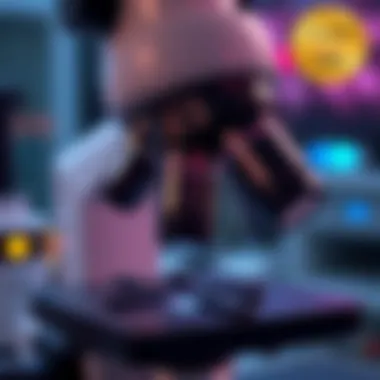
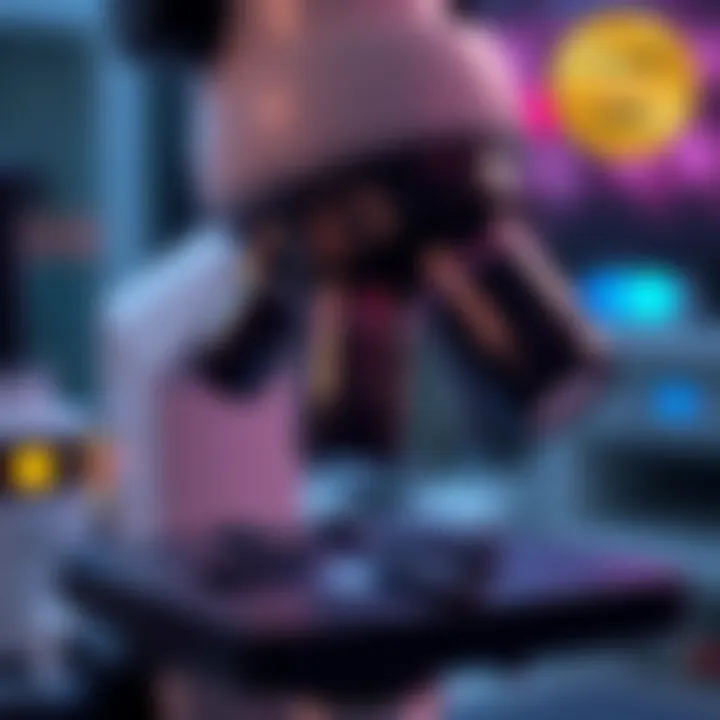
Intro
Optical metallurgical microscopes are incredible instruments that combine precision optics with intricate details of material structures. At the intersection of physics, engineering, and materials science, these devices allow researchers and professionals to explore the micro-world of metals and alloys. Understanding their design, operation, and applications is pivotal for anyone involved in metallurgy or materials research.
The journey into the realm of optical metallurgical microscopy begins with a clear understanding of its core principles. These microscopes are not just tools; they breathe life into the microscopic structures that form the foundation of materials we encounter in daily life. From the steel beams in skyscrapers to the wires in our electronics, every metal has a story to tell, and these microscopes help us narrate that tale through detailed analysis.
The importance of optical metallurgical microscopes stretches beyond mere academic interest. They have become indispensable in industries where understanding material properties is crucial for safety, efficiency, and innovation. Through their capability to magnify tiny sample features, these instruments shed light on microstructural characteristics, aiding in defect analysis and material optimization.
Key Concepts
Definition of the Main Idea
Optical metallurgical microscopes serve as a gateway to the intricate world of materials at the micro level. These microscopes typically utilize light to enhance the visibility of small structures within metal samples. They allow scientists to observe and document crystallographic features, grain boundaries, and various phases within a sample. This examination is vital for understanding how microscopic properties influence macroscopic behavior.
Overview of Scientific Principles
The design and functionality of optical metallurgical microscopes are grounded in several scientific principles:
- Optics: The way light interacts with materials is fundamental. Lenses bend light rays to magnify images, allowing detailed observations of microstructures.
- Sample Preparation: Proper sample preparation is crucial. It involves cutting, grinding, polishing, and etching samples to reveal structures without introducing artifacts.
- Contrast Methods: Various techniques, such as bright field, dark field, and polarized light microscopy, aid in enhancing features of interest.
"The intricacies of microscopy bring to light the often-overlooked details that define material behaviors and applications."
"The intricacies of microscopy bring to light the often-overlooked details that define material behaviors and applications."
Current Research Trends
Recent Studies and Findings
In recent years, significant advancements have been made in the field of optical metallurgical microscopy. Notably, studies focusing on automated image analysis using artificial intelligence have gained momentum. Automation in capturing and analyzing images enhances accuracy and efficiency, reducing human error.
- Research indicates a shift toward real-time analysis, helping in rapid identification of defects during production.
- Investigations into nano-scale features have expanded the utility of these microscopes in identifying new material composites.
Significant Breakthroughs in the Field
One remarkable breakthrough has been the integration of 3D microscopy techniques into traditional optical systems. This advancement provides a more holistic view of materials, allowing for depth and structural assessment.
Additionally, enhancing digital platforms for data sharing creates opportunities for collaborative research. Scientists now can exchange observations in real-time, fostering a collective advancement of knowledge in materials science.
As we dive deeper into the subsequent sections, expect a thorough examination of how optical metallurgical microscopes not only shape our understanding of materials but also influence the future of various industries.
Prelude to Optical Metallurgical Microscopes
Optical metallurgical microscopes, a cornerstone of material science, serve a very specific yet crucial purpose in examining the microstructure of various materials. Their importance can hardly be understated; they provide insights into the intricacies of materials that are otherwise invisible to the naked eye. Understanding these microstructural details is pivotal for industries that rely on the integrity and quality of their materials, such as metallurgy, manufacturing, and materials engineering.
The ability of these microscopes to highlight defects, grain structures, and phases within materials is invaluable when it comes to quality assessment and R&D. In a world where materials are becoming increasingly sophisticated, having the tools that aid in high-precision analysis is not just beneficial—it is essential.
Furthermore, optical metallurgical microscopes combine impressive optical principles with user-friendly design, making them a favored choice among researchers, engineers, and educators alike. They allow for real-time observation and documentation, which is critical for both academic studies and practical applications in industry.
"The microscope is a window to the microscopic world and offers revelations that often lead to groundbreaking discoveries in materials research."
"The microscope is a window to the microscopic world and offers revelations that often lead to groundbreaking discoveries in materials research."
Definition and Purpose
At its core, an optical metallurgical microscope is designed specifically for examining the physical structure and details of metals and other materials. The intent is not merely to see larger-than-life images but to comprehend the nuanced characteristics of these materials. This includes the evaluation of properties like hardness, tensile strength, and fatigue resistance, which critically depend on the microstructural constitution of materials.
The microscope utilizes visible light and a series of optical components, allowing researchers to magnify and observe samples in great detail. It serves numerous purposes, from basic educational demonstrations to advanced laboratory analysis. Being versatile, optical metallurgical microscopes can handle a wide array of materials, including metals, polymers, and ceramics—each with its own microscopic features that can influence overall performance.
Historical Overview
The journey of optical metallurgical microscopy is as fascinating as the technology itself. The roots trace back to the late 19th century when there was a growing need for detailed investigations into metal structures, notably during the industrial revolution. Pioneering metallurgists recognized that understanding material behavior under different conditions could lead to developments in durability and quality.
Early instruments were rather rudimentary, but they paved the way for innovations that flourished in the 20th century. With the invention of new optical technologies, such as phase contrast microscopy and polarized light microscopy, the field advanced significantly. Such technologies dramatically improved the clarity of images and the types of analyses that could be conducted.
Innovations in sample preparation techniques also played a critical role, as they enhanced the ability of microscopists to view and analyze specimens effectively. Today’s models are a culmination of centuries of development, integrating advanced optics with flexible, often digital, imaging processes that push the boundaries of what can be observed.
In summary, understanding the evolution of optical metallurgical microscopes provides valuable context for their current applications and capabilities, reinforcing their place as essential tools in the field of materials science.
Fundamental Principles of Optics
Understanding the fundamental principles of optics is crucial for grasping how optical metallurgical microscopes function. The behavior of light, its interaction with various materials, and the optical components employed are the cornerstone of effective microscopy. Each principle influences the microscope's ability to provide detailed and accurate images of microstructures, making it a key area of focus in this examination.
Light Behavior and Interaction
Light, at its core, is an electromagnetic wave that behaves in unique ways depending on the environment it encounters. When it strikes a material, several phenomena can occur—reflection, refraction, and absorption, to name a few.
- Reflection: This happens when light bounces off a surface. It's a principle we often observe in daily life, like seeing our faces in a mirror.
- Refraction: This describes how light bends when passing from one medium to another. A classic example is a straw appearing bent when placed in a glass of water. In microscopy, refraction is vital as it allows for magnification.
- Absorption: Certain materials absorb specific wavelengths of light, which can alter the color and clarity of what we see under the microscope.
These behaviors determine how effectively a microscope can capture the intricate details of a sample. Understanding these interactions helps materials scientists and metallurgists interpret the microstructural properties of materials accurately.
Optical Components Overview
The optical components of a metallurgical microscope are the gears that drive its functionality. Various parts work in tandem, allowing for precision and clarity in imaging. Key components include objective lenses, eyepieces, and optical filters.
Objective Lenses
Objective lenses are arguably the heart of the microscope's imaging system. They gather light from the specimen and form a magnified image. These lenses come in various types, such as achromatic, planachromatic, and apochromatic, each with distinct characteristics. A significant aspect of objective lenses is their numerical aperture (NA), which relates to the lens's ability to gather light and resolve fine specimen detail.
Key Characteristic: The numerical aperture is essential for high-resolution imaging. A higher NA allows for better resolution, enabling scientists to observe finer details in the microstructure with increased clarity.
Unique Feature: Apochromatic lenses correct for chromatic and spherical aberration, enhancing image quality significantly. The downside? They tend to be more expensive than simpler lens types, which may be a consideration for smaller labs.
Eyepieces
Eyepieces serve as an interface between the microscope and the observer's eye. They amplify the image produced by the objective lens for even greater detail. It's common to see eyepieces rated at 10x or 15x magnification. One critical aspect of eyepieces is the field of view (FOV), which is the area visible through the microscope.
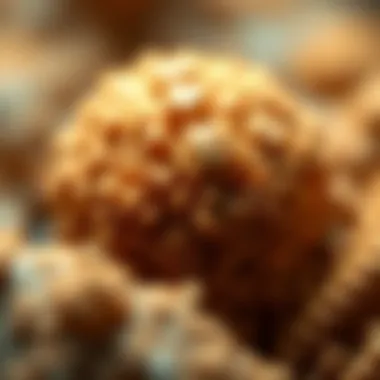
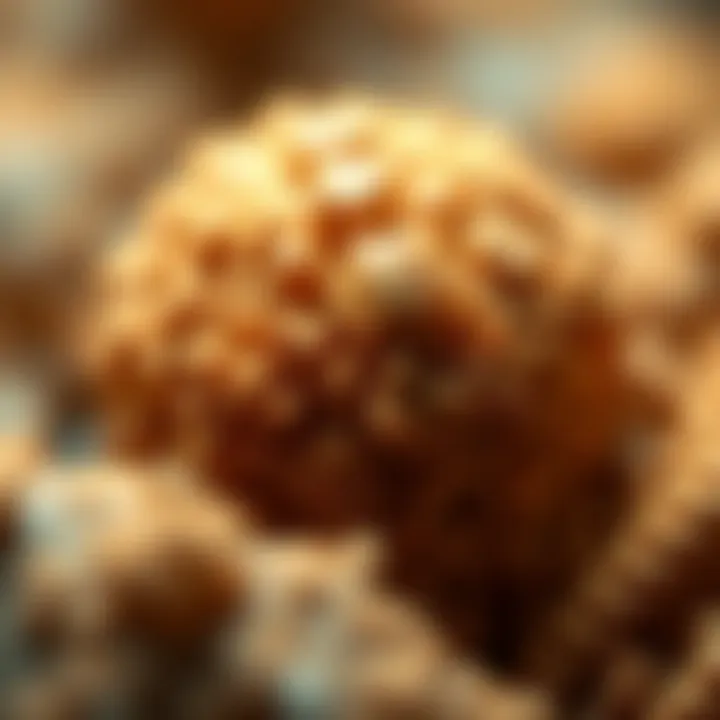
Key Characteristic: A wider FOV allows for easier viewing of larger specimens or multiple samples at once. This feature is particularly beneficial when working with complex materials that require a holistic view.
Unique Feature: Some eyepieces incorporate reticles, which can aid in measuring the size of structures being observed, although this can complicate the viewing experience for those unfamiliar with such tools.
Optical Filters
Optical filters play a pivotal role in controlling the light that reaches the specimen. They can enhance contrast, suppress specific wavelengths, or allow only certain colors to pass through, thereby improving image quality. Filters can be critical while observing samples with varying degrees of transparency, such as in fluorescence microscopy.
Key Characteristic: Selective wavelength transmission is a key benefit. For instance, using a bandpass filter can allow only the relevant wavelength range needed for a specific analysis, enhancing observed details.
Unique Feature: Some filters can also assist in reducing glare, which is useful when viewing shiny metallic surfaces that might otherwise obscure important details. However, the use of filters can sometimes introduce extra steps during sample preparation.
In summary, these fundamental principles and components of optics facilitate a deeper understanding of materials through microscopy. The interplay of light and optical systems serves to transform the microscopic world into an observable reality, paving the way for advancements in materials science and beyond.
Design Features of Optical Metallurgical Microscopes
The design features of optical metallurgical microscopes play a pivotal role in their functionality and effectiveness in materials science. A thorough understanding of these aspects can significantly enhance the user experience and the insights drawn from microscopic examinations. These instruments must not only possess high-quality optics but also ergonomic design and flexibility in their configurations. Each facet of design, from construction material to illumination methods, contributes to the microscope's overall performance and the quality of the observations.
Construction and Configuration
The construction of an optical metallurgical microscope is a blend of engineering precision and optical innovation. Typically, these microscopes include a robust frame that minimizes vibrations, ensuring stability during use.
An important feature of construction is the choice of materials. High-grade metals and composites are preferred for durability and weight distribution. In terms of configurations, modular designs are becoming increasingly favored, allowing users to swap components—like lenses and illumination sources—according to their specific needs. This adaptability ensures that a single microscope can serve various purposes, adapting efficiently to different research tasks or industrial applications.
Illumination Techniques
Illumination techniques are crucial in determining the clarity and detail visible through the microscope. Different methods can drastically alter how samples are perceived, each having unique benefits and challenges.
Brightfield
Brightfield microscopy is perhaps the most straightforward of the illumination techniques. This approach illuminates the specimen from below, allowing light to pass through the sample, making it appear bright against a darker background. It is a fundamental technique, serving as a stepping stone for many other methods. One unique characteristic of brightfield is its simplicity—no special preparations are needed for most samples. This ease of use makes it popular in educational settings and among beginners wanting to explore materials without extensive background knowledge.
However, brightfield does have limitations. It often fails to highlight subtle differences in materials with similar refractive indices, which can lead to the overlooking of critical details in microstructural analysis.
Differential Interference Contrast
Differential interference contrast (DIC) microscopy enhances specimens' contrast by utilizing polarized light and a specialized optical setup. A significant aspect of DIC is its ability to produce images with a three-dimensional feel and enhanced edge detection. This characteristic makes it particularly valuable in biological applications, but it also sees extensive use in metallurgy for studying surface textures and layer behaviors.
While DIC offers superb contrast and depth, it requires careful calibration of the optical components. This added complexity might deter some users but yields rewarding insights when executed correctly.
Fluorescence
The fluorescence technique stands as a sophisticated method that relies on the sample fluorescing under specific wavelengths of light. The key characteristic of fluorescence microscopy is its ability to visualize structures that would otherwise be invisible under standard light, thanks to the targeted use of fluorescent dyes.
One significant advantage of fluorescence is its ability to provide high-resolution images of specific components in complex materials. It can distinguish between different phases or impurity levels in metals, facilitating deeper analysis. However, this method does depend heavily on adequate sample preparation and the use of specific light sources, which can add to the complexity and time required during the preparation stage.
Optical techniques like fluorescence enable researchers to delve deep into material characteristics, unveiling details critical for advancements in science and industry.
Optical techniques like fluorescence enable researchers to delve deep into material characteristics, unveiling details critical for advancements in science and industry.
In summary, understanding the various illumination techniques—brightfield, differential interference contrast, and fluorescence—equips professionals with the tools necessary for detailed analysis in metallurgy. Each technique complements the others, helping to provide a fuller picture of the microscopic world and the properties of materials being studied.
Applications in Materials Science
The role of optical metallurgical microscopes in materials science cannot be overstated. These instruments are fundamental tools for understanding not only the physical properties of materials but also their structural flaws and overall integrity. In the realm of materials science, precise examination at the microstructural level offers invaluable insights, paving the way for advancements in engineering, quality control, and innovation in material development. Here are a few of the specific elements that highlight their importance:
- Microstructural assessment: Knowledge of microstructures plays a crucial role in determining how materials behave under different stresses. Identifying microstructural features helps in predicting the performance and longevity of materials.
- Material selection and improvement: Understanding the microstructural characteristics allows scientists and engineers to select or modify materials for better performance in specific applications.
- Quality assurance: Regular inspection via optical metallurgical microscopes helps ensure that products meet industry standards and specifications, thereby reducing defects and enhancing product reliability.
Microstructural Analysis
Microstructural analysis serves as the backbone of materials science where optical metallurgical microscopes shine. They provide an in-depth look at grain size, phase distributions, and the presence of impurities within a sample. Each of these aspects contributes significantly to the mechanical properties of materials, influencing their strength, ductility, and hardness. Effective microstructural assessment can also offer insights into processing conditions and thermal treatments endured by materials during manufacturing.
In practical terms, microscopes facilitate the evaluation of features such as:
- Grain Boundaries: These boundaries can affect a material’s conductivity and mechanical properties.
- Phase Composition: Changing phases in materials have far-reaching implications on performance, influencing applications from aerospace to civil engineering.
By discerning subtle changes in microstructures, metalurgists are equipped to refine existing materials or innovate new ones.
Phase Identification
Phase identification is another critical aspect that can’t be overlooked when discussing optical metallurgical microscopy. This task entails recognizing the various phases present in a material and understanding how they relate to each other. Knowing the phases in a material can inform choices regarding processing and applications, directly impacting quality and performance.
Grain Structures
Grain structures are integral to analyzing the microstructure of materials. They refer to the arrangements and sizes of individual crystal grains within a material and can affect its overall behavior. One of the key characteristics of grain structures is their ability to dictate mechanical properties like strength and ductility. For instance, fine-grained materials often exhibit superior strength compared to coarse-grained ones. This makes grain structures particularly valuable in studies focused on optimizing material performance.
Additionally, grain structures are beneficial choices for researchers seeking to link manufacturing processes with material performance. For those developing new alloys or composites, analyzing grain structure can reveal specific ways processing parameters impact the final properties.
However, one unique feature of grain structures is their response to thermal treatments. An advantage here is that by manipulating grain size through controlled heating, metallurgists can tailor properties as desired. The downside is that achieving the ideal grain size can be a tricky exercise — too fine a grain may lead to brittleness while coarser grains can reduce toughness.
Phase Diagrams
Phase diagrams serve as a crucial tool for visualizing the relationship between different phases in a given material under various conditions of temperature and composition. A distinctive aspect of phase diagrams is that they outline the conditions under which a specific phase is stable, which is essential for making practical decisions in metallurgy.
These diagrams often provide valuable insights into aspects such as solubility limits and the resultant microstructures that can form during cooling. Their key characteristic lies in their ability to provide a blueprint not only for current material states but also for predicting the effects of future treatments.
Phase diagrams are a popular choice among researchers because they can guide alloy development and processing. The unique feature of phase diagrams is their predictive power; understanding how different phases interact allows for better control over material properties. However, their disadvantage manifests when considering complex systems where multiple phases can appear, complicating interpretations and requiring sophisticated knowledge to navigate effectively.
Understanding microstructural and phase characteristics is essential for advancing materials science and enhancing technological applications.
Understanding microstructural and phase characteristics is essential for advancing materials science and enhancing technological applications.
Sample Preparation Techniques
In the arena of optical metallurgical microscopy, the significance of sample preparation cannot be overstated. Proper preparation serves as the bedrock for obtaining reliable and high-quality microscopic images. Without meticulous sample preparation, a researcher may misinterpret microstructural features, leading to erroneous conclusions and potential pitfalls in further analysis. Therefore, understanding the specific techniques applicable to sample preparation is paramount.
Material Mounting
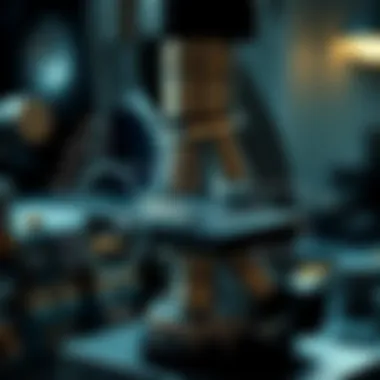
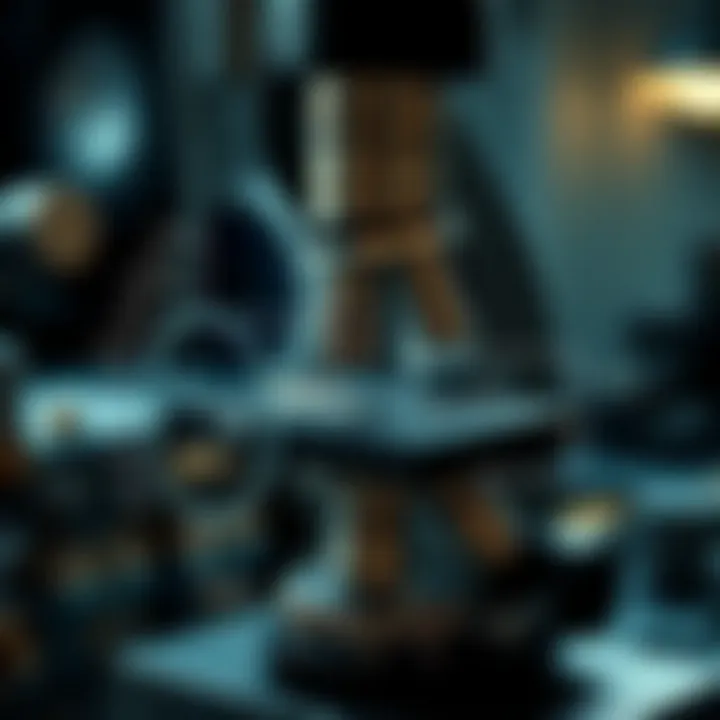
Material mounting involves fixing a specimen securely in a medium suitable for polishing and etching. The choice of mounting material may vary based on the properties of the sample and the requirements of subsequent analysis. For instance, hot mounting is often preferred for thermoplastics, while cold mounting can suffice for heat-sensitive samples. The end goal remains clear: to secure the material in a way that facilitates further processing without introducing artifacts.
When choosing mounting materials, the compatibility with the specimen is crucial. Mounting resins, epoxy, or acrylics are often used; each of these options offers unique benefits. For example, epoxy resins are typically more robust, providing excellent mechanical stability, while acrylics allow for easier specimen identification due to their transparency. Regardless of the method chosen, a well-prepared mount can dramatically improve subsequent polishing and observation results.
Polishing Techniques
Proper polishing techniques play a vital role in preparing the surface of the specimen for examination. Different methods can yield significantly different results in terms of flatness and surface roughness; therefore, understanding the nuances of polishing is essential.
Mechanical Polishing
Mechanical polishing is one of the most popular methods used in sample preparation due to its effectiveness at achieving a high-quality finish. In this technique, rotating discs coated with abrasives gradually remove material from the surface of the specimen. A key characteristic of mechanical polishing is the use of varied grit sizes, allowing for a tailored approach to remove scratches and imperfections progressively.
What's beneficial about mechanical polishing is its relatively straightforward execution. It allows for a controlled process where the operator can adjust variables like speed and pressure to optimize results. However, special care must be taken as excessive polishing can damage the specimen—potentially leading to subsurface deformation. To avoid this, it is advisable to monitor the process closely and use optimal pressure settings.
Electropolishing
Electropolishing, on the other hand, represents a more technologically advanced polishing method. In this electrochemical process, an electric current is passed through an electrolyte solution, leading to the removal of the surface material from the specimen. The crucial factor that sets electropolishing apart is its ability to leave the surface very smooth without risking the introduction of scratches, a common issue with mechanical polishing.
The high degree of cleanliness and the uniformity of the finish achieved through electropolishing make it a favored choice for many metallurgical applications. However, it requires precise control of parameters such as current density and electrolyte composition, making it a less approachable method for some. Additionally, the process can be somewhat time-consuming and may necessitate a deeper understanding of the chemical reactions involved, which may pose a barrier for certain practitioners.
"The choice of polishing technique can decisively affect the outcome of the microscopy process, impacting everything from image clarity to the accuracy of microstructural analysis."
"The choice of polishing technique can decisively affect the outcome of the microscopy process, impacting everything from image clarity to the accuracy of microstructural analysis."
Ultimately, selecting the appropriate polishing technique depends on various factors, including the materials involved and the specific objectives of the microscopy examination. Achieving an optimal surface finish not only enhances image quality but also provides more reliable data on the microstructural characteristics of the sample.
Quantitative and Qualitative Analysis
The realm of optical metallurgical microscopes offers a rich tapestry of quantitative and qualitative analyses crucial for deciphering material microstructures. These two analytical approaches, while different in nature, work hand-in-hand to deliver a more rounded understanding of metallurgical phenomena. Their importance cannot be overstated, as both methods contribute significantly to research advancements and practical applications in materials science.
Quantitative analysis is akin to looking through a microscope to gather hard numbers. It focuses on measuring specific properties of the sample, like grain size, phase distribution, and porosity. This type of analysis is vital for
- Material characterization
- Quality assurance
- Understanding failure mechanisms
Quantitative data provides a solid foundation for comparing different materials and processes under various conditions. When researchers can put numeric values to their observations, it allows for more rigorous statistical analyses and stronger conclusions drawn from experiments.
On the other hand, qualitative analysis dives into the nuances. It emphasizes understanding the structure, composition, and morphology of materials without the heavy reliance on numerical data. This approach helps in recognizing the relationships between the microstructure and the physical properties of the material.
This duality in analysis paves the way for comprehensive investigations involving:
- Microstructural imaging
- Phase identification
- Visual interpretation of defects
By leveraging both quantitative and qualitative analyses, scientists are better equipped to interpret complex data sets, leading to richer insights into materials behavior under various conditions.
Image Capture and Processing
In the domain of optical metallurgical microscopy, image capture and processing stands as a pillar of both qualitative and quantitative analysis. It’s not just about taking pretty pictures of samples; it’s about capturing the essence of the materials being studied. The quality of images directly relates to the accuracy and reliability of the analyses performed later on. High-resolution imagery allows researchers to observe intricate details that are essential for thorough analyses.
Processing these images involves a host of software tools that can enhance, transform, and analyze the images for better clarity and quantitative measurements. Techniques such as image segmentation, filtering, and feature extraction play pivotal roles here.
Data Interpretation
The interpretation of data gathered from optical metallurgical microscopes hinges significantly on the methodologies employed in both qualitative and quantitative analyses. Proper data interpretation can substantiate or overturn theoretical models, enhancing our understanding of material behaviors.
Statistical Methods
Statistical methods offer a structured framework for analyzing data sets derived from microscope observations. They stand out because they facilitate the identification of trends, correlations, and deviations in material properties. One key characteristic of statistical methods is their ability to provide confidence levels and variability assessments—an invaluable aspect when validating findings. They are often deemed beneficial because:
- They assist in making sense of complex data.
- They enable comparisons between different studies or experiments.
One unique feature of statistical methods is their use of hypothesis testing, which can determine whether findings are statistically significant. However, a potential disadvantage lies in their reliance on sample sizes; small or biased samples can lead to misleading conclusions.
Software Applications
In this modern age, the role of software applications in optical microscopy cannot be overlooked. These applications help streamline workflows and enhance data interpretation. The key characteristic of software applications tailored for microscopy is their analytical capabilities, often integrating sophisticated algorithms for image processing and quantitative analysis.
They are especially popular as they allow users to manipulate images and data without needing extensive programming knowledge. Some notable advantages include:
- Automation of mundane tasks
- Enhanced accuracy in measurements
Yet, one must remain cautious; sometimes, software may produce results that require human interpretation to ensure that analytical outcomes align with real-world observations. Unconventional results should prompt further investigation beyond the numbers.
Understanding both quantitative and qualitative analysis through proper image capture and data interpretation is critical for progress in materials science and related fields.
Understanding both quantitative and qualitative analysis through proper image capture and data interpretation is critical for progress in materials science and related fields.
Advanced Technologies in Microscopy
In the ever-evolving landscape of microscopy, advanced technologies play a pivotal role in augmenting our ability to understand and analyze materials at microscopic levels. The field has transitioned from traditional methods to cutting-edge techniques that enhance image quality, provide deeper insights into microstructural properties, and offer new dimensions of analysis. Part of this advancement comes through the integration of digital tools that allow for more precise measurement and interpretation, thus opening doors to fresh scientific inquiry.
Understanding advanced technologies in microscopy is vital for students, researchers, and professionals alike. It is not merely about the sophisticated equipment but rather how these advancements transform theoretical frameworks into practical applications. The integration of these technologies contributes significantly to both academic research and industrial applications.
Confocal Microscopy
Confocal microscopy is a standout technology in this domain, allowing for the capture of high-resolution images while eliminating out-of-focus light. This technique employs a pinhole to block out light that does not originate from the focal plane of the specimen, resulting in vastly improved contrast and resolution. This clarity enables researchers to visualize fine details within the sample that they might miss with traditional light microscopy.
Key benefits of confocal microscopy include:
- Enhanced Resolution: With its ability to optically section samples, confocal microscopy achieves better resolution compared to standard microscopes.
- Three-Dimensional Imaging: This technique supports the generation of 3D images from 2D slices, providing a comprehensive view of the sample.
- Live Cell Imaging: Researchers can observe dynamic processes within living cells, gaining insights that are critical for studies in cell biology and developmental biology.
- Multicolor Imaging: The use of multiple fluorescent dyes enables simultaneous observation of different structures within the same sample.
Despite its advantages, confocal microscopy comes with considerations such as:
- Cost: The equipment tends to be expensive, which could be a barrier for some institutions.
- Complexity: Operating confocal microscopes requires specialized training to interpret data accurately.
Digital Microscopy
Digital microscopy represents another breakthrough, merging traditional optical techniques with digital imaging technology. Here, the specimen's images are captured digitally, enabling immediate enhancement, analysis, and sharing without the need for film development. This approach streamlines workflows across various applications, making it a favored method in both educational and industrial contexts.
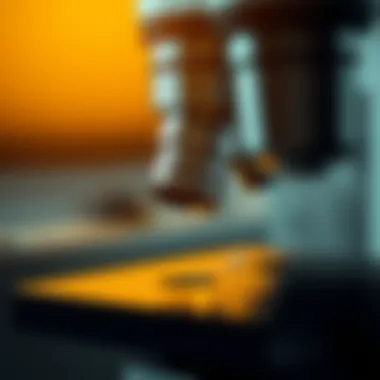
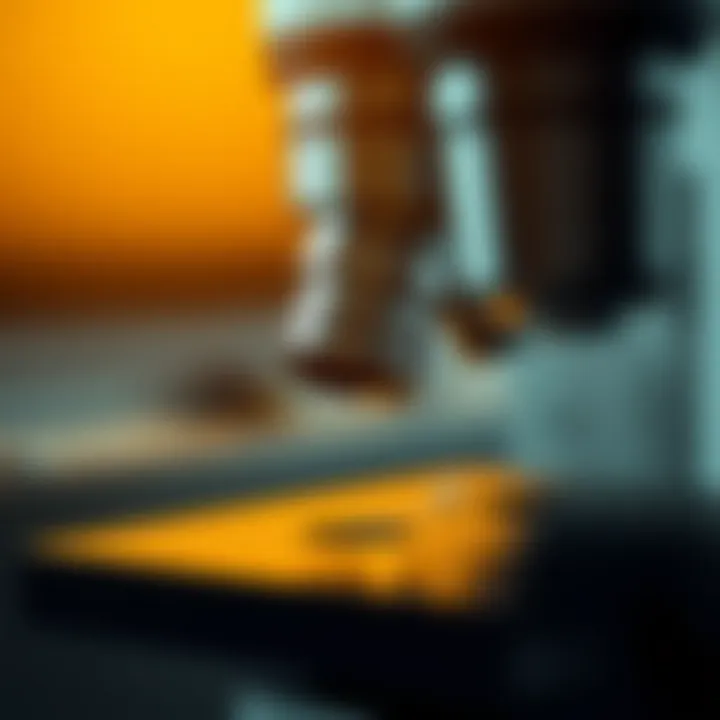
The merits of digital microscopy include:
- Instant Analysis: Researchers can view and analyze images in real-time, leading to quicker decision-making.
- Data Storage and Organization: Digital images are easier to store, retrieve, and organize than physical slides.
- Remote Collaboration: With digital files, sharing results with colleagues across the globe is seamless.
- Advanced Software Integration: The use of sophisticated software for image analysis allows for more in-depth examination, such as statistical analysis or automated measurements.
Yet, digital microscopy is not without its drawbacks:
- Equipment and Software Dependence: Successful application depends on having the right equipment and software capabilities, which might not be universally available.
- Learning Curve: Users might require guidance to navigate the software effectively, especially those new to digital techniques.
The blend of advanced methodologies like confocal and digital microscopy paves the way for revolutionary developments in materials sciences and other research fields. By continually adapting to these technologies, optical metallurgical microscopes remain at the forefront of exploration and discovery, making an indelible impact on contemporary research and industrial practices.
The Role of Optical Metallurgical Microscopes in Industry
Optical metallurgical microscopes play a vital role in various industries, especially those related to materials science and metallurgy. These instruments provide a window into the microscopic world of materials, enabling scientists and engineers to observe and analyze microstructures. Without these microscopes, activities such as quality control, research, and product development would be exceedingly complex and likely less effective.
Quality Control Processes
In the realm of quality control, optical metallurgical microscopes are indispensable. Manufacturing industries rely on these tools to ensure that materials meet specified standards and perform reliably in their intended applications. One of the main advantages of using these microscopes in quality control is their ability to identify surface irregularities and structural flaws at a microscopic level. For example, during the production of steel, these microscopes can reveal grain structures that might indicate weaknesses.
Some key benefits of incorporating optical metallurgical microscopes into quality control processes include:
- Detection of Defects: By offering high-resolution images, they can help identify micro-defects that might not be visible to the naked eye.
- Consistency Assessment: They enable the comparison of microstructural features among batches of materials, assuring that they adhere to quality specifications.
- Improved Feedback: The immediate feedback loop created by using these microscopes allows manufacturers to adjust processes on the fly, rather than relying solely on post-production evaluations.
"In today's fast-paced industrial environment, having the ability to assess the integrity of materials rapidly can mean the difference between success and failure in production."
"In today's fast-paced industrial environment, having the ability to assess the integrity of materials rapidly can mean the difference between success and failure in production."
Research and Development
Furthermore, optical metallurgical microscopes are essential tools in research and development (R&D). Scientists depend on these microscopes to explore new materials, optimize manufacturing processes, and develop innovative solutions to engineering challenges. In R&D settings, the microscopes serve various functions, from experimental examination to collaborative studies.
In R&D, the benefits include:
- Material Innovation: The ability to analyze the microstructure at the atomic level can lead to the discovery of new materials or enhancements to existing ones, fostering innovation.
- Process Optimization: Understanding the behavior of materials under different conditions assists in fine-tuning processing techniques, thus improving efficiency and yielding better end products.
- Validation of Theories: Researchers can use data collected from optical metallurgical microscopes to validate or challenge existing theoretical frameworks, paving the way for advancements in materials science.
In summary, the role of optical metallurgical microscopes in industry is multifaceted, impacting both quality control and the advancement of research. Their integration into these processes not only improves accuracy and efficiency but also aids in the innovation of materials and methods, significantly influencing industrial progress.
Challenges and Limitations
In the realm of optical metallurgical microscopes, acknowledging the challenges and limitations is paramount for both practitioners and researchers. These instruments, while powerful, come with their set of hurdles that can significantly affect the analysis and interpretation of microstructural properties. Understanding these challenges not only aids in effective utilization but also informs decisions in product selection, design modifications, and educational approaches.
Technical Challenges
The complexity of optical metallurgical microscopes often leads to various technical challenges that can impede their efficiency. For instance, the alignment of optical components such as objective lenses and eyepieces is crucial for obtaining optimal imagery. A slight misalignment can lead to aberrations, distorting the observed structure and potentially skewing results.
Moreover, the selection and adjustment of illumination techniques, whether brightfield, fluorescence, or differential interference contrast, can present significant difficulties. Each method requires specific configurations and calibration to meet analysis standards.
Handling sample preparation can also pose a challenge, where improper mounting or polishing could alter sample characteristics and complicate observations. All these factors contribute to a higher learning curve for users, especially those new to metallurgy or microscopy in general.
"The mastery of optical metallurgical microscopes lies not only in their operation but also in understanding and mitigating various technical pitfalls."
"The mastery of optical metallurgical microscopes lies not only in their operation but also in understanding and mitigating various technical pitfalls."
Cost Considerations
Cost is another critical factor that cannot be overlooked when discussing optical metallurgical microscopes. These sophisticated instruments often come with a hefty price tag, which can be daunting for educational institutions or smaller research facilities. The upfront costs can be a barrier to entry, limiting access to high-quality imaging for many budding metallurgists and researchers.
Also, there are ongoing costs associated with maintenance and the procurement of necessary accessories and consumables. Expensive objective lenses, specialized filters, and replacement bulbs contribute to a cumulative financial burden. In some cases, the cost of proper training to effectively use these microscopes also adds to their overall expense.
Therefore, institutions must evaluate the potential return on investment in these microscopes versus the necessity based on research demands. Balancing quality and budget is critical since settling for a lower-quality microscope can lead to subpar results and, in turn, affect credibility in research findings.
In essence, both technical challenges and cost considerations play a significant role in shaping the landscape of optical metallurgical microscopy, thereby influencing future innovations and applications within the field.
Future Trends in Optical Microscopy
The evolution of optical microscopy is not just a tale of technological advancements; it encapsulates the dynamic nature of scientific inquiry and the relentless pursuit for precision in the microscopic domain. With the ongoing metamorphosis in this field, several trends are emerging, shaping both the tools and techniques employed by researchers today. Recognizing these shifts is crucial for educators, students, and professionals involved in materials science and metallurgy, as it will prepare them for the future landscape of their work.
Emerging Technologies
A significant trend in optical microscopy is the advent of cutting-edge technologies that enhance imaging capabilities and offer greater flexibility in applications. Here are some notable advancements:
- Super-resolution Microscopy: This tech defies the diffraction limit of light, allowing researchers to visualize structures at molecular dimensions. Techniques like STED (Stimulated Emission Depletion) and PALM (Photo-Activated Localization Microscopy) permit unprecedented detail, making it invaluable for studying materials science at a microstructural level.
- Adaptive Optics: Originally developed for astronomy, this approach adjusts optical systems in real-time to correct distortions. By utilizing adaptive optics, meso-scale imaging becomes clearer and more accurate, providing a clearer view of surface structures and defects in metallurgical specimens.
- Automated Imaging Systems: Automation is becoming a key player in microscopy, enabling high-throughput analysis. Instruments equipped with automated stage movements and real-time image processing can significantly accelerate data collection while minimizing human error.
"The future of microscopy lies not only in better optics but also in the synergy between automation and advanced algorithms."
"The future of microscopy lies not only in better optics but also in the synergy between automation and advanced algorithms."
Integration with Other Analytical Techniques
As microscopy continues to evolve, its integration with other analytical techniques is on the rise, offering a multidisciplinary approach to materials analysis:
- Spectroscopy Coupling: Techniques such as Fourier Transform Infrared Spectroscopy (FTIR) or Raman Spectroscopy can be coupled with optical microscopy to provide chemical composition information alongside microstructural insights. This combined approach is particularly beneficial in identifying phases and analyzing grain boundaries in metals and ceramics.
- Electron Microscopy Integration: The synergy of optical and electron microscopy is particularly beneficial for detailed surface and elemental analysis. While optical microscopy provides a broad view, electron microscopy can give atomic-level insights. These complementary methodologies enable robust material characterization, leading to a deeper understanding of material properties.
- Data Fusion and Machine Learning: As the amount of data generated through microscopy steadily increases, utilizing machine learning algorithms and data fusion techniques is becoming essential. By correlating data from various sources, like optical and electron microscopy, researchers can derive more meaningful insights into material behaviors and properties.
In summary, the future of optical microscopy is poised for radical improvements through emerging technologies and their increased integration with other analytical tools. For students and professionals alike, keeping abreast of these trends is not merely beneficial; it’s essential for making informed decisions in research and application.
As the landscape of optical microscopy transforms, embracing these advancements will guide the next generation of metallurgical and materials research. The implications stretch beyond mere instrument improvements—they signify a holistic enhancement in the understanding of materials that will resonate throughout various scientific domains.
End
In the realm of optical metallurgical microscopes, the conclusion serves as an essential reflection on the intricate web of information woven throughout the article. The understanding of optical metallurgical microscopes is not merely a quest for knowledge but a crucial stepping stone in multiple fields, particularly in materials science and metallurgy. Their ability to reveal the microstructural secrets embedded within various materials cannot be overstated.
Summary of Key Points
As this article draws to a close, several key points leap to the forefront:
- Definition and Purpose: Optical metallurgical microscopes provide unparalleled views into the microstructures of metals and other materials, aiding in the identification and analysis of composition and properties.
- Historical Context: A look back at the development of these instruments reveals a progression fueled by technological advancements, making them vital tools in both research and industry.
- Technical Features: The design elements, including various illumination methods such as brightfield and fluorescence, contribute to the performance and versatility of these microscopes.
- Applications: The discussion on applications highlights their role in microstructural analysis and phase identification, reinforcing their importance in quality control processes within various industries.
- Future Trends: Emerging technologies such as confocal and digital microscopy showcase the potential for enhanced capabilities, predicting a bright future for optical metallurgical microscopy.
Implications for Future Research
The implications of this exploration extend well beyond the boundaries of current understanding. Future research should consider:
- Integration with Advanced Technologies: The merging of optical techniques with other analytical methods can open new avenues for discovery, enhancing the depth of analysis possible with these systems.
- Educational Curriculum Enhancements: As industries increasingly rely on these technologies, incorporating in-depth lessons on optical microscopy into academic programs will better prepare future professionals.
- Innovation in Sample Preparation: Research into refining sample preparation techniques may yield better results, allowing for more accurate interpretations of data obtained from optical metallurgical microscopes.
In sum, the conclusion not only encapsulates vital points discussed but also inspires a look forward. As technologies evolve, the importance of mastering optical metallurgical microscopes will only grow, making this topic critically relevant for all stakeholders involved.