Exploring the Topo Cloning Protocol in Molecular Biology
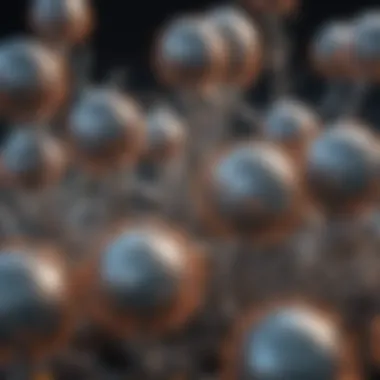
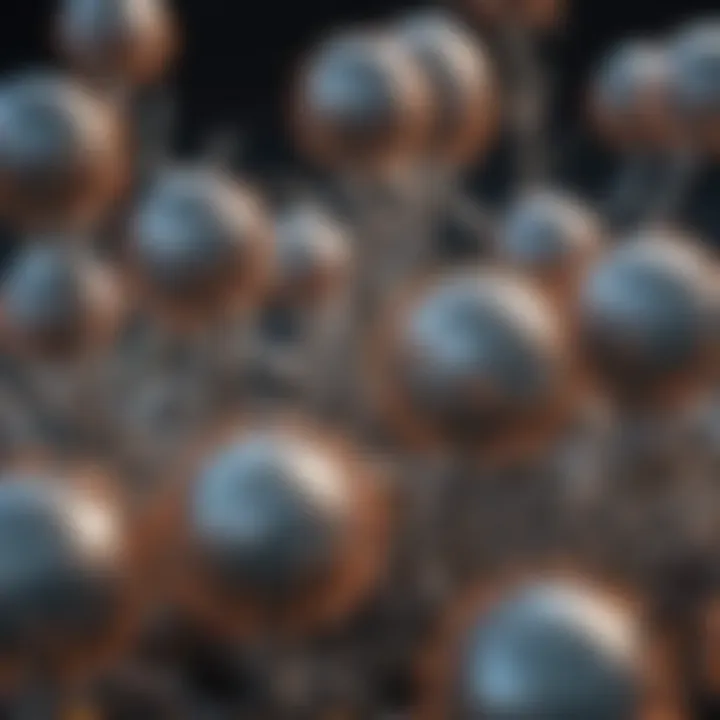
Intro
Molecular biology has a vast toolkit, and one of its cornerstones is the topo cloning protocol. A key technique that allows researchers to manipulate DNA with precision, topo cloning has become a go-to method for creating recombinant DNA. Whether you're in a lab studying gene functions or working on developing new therapeutic agents, understanding topo cloning can elevate your experiments.
What makes topo cloning so intriguing is its efficiency. Traditional cloning methods, while effective, can often be cumbersome and time-consuming. Here enters topo cloning, which employs topoisomerases to facilitate the insertion of DNA fragments into vectors. Depending on the specific requirements, this protocol can save significant time and resources.
With this in mind, our exploration of topo cloning will not just skim the surface. We will delve into its fundamental concepts, recent advancements, and practical applications—all crafted to sharpen your molecular biology skills.
> "The beauty of topo cloning is its versatility, making it a mainstay for those venturing into the intricate world of genetic engineering."
So buckle up as we navigate through the essential aspects of topo cloning, from its core principles to the latest developments in the field.
Preamble to Topo Cloning
The field of molecular biology is continuously evolving, shaping our understanding of genetic material and offering innovative techniques to manipulate it. Topo cloning stands out as a powerful approach, intricately designed to streamline the cloning process. As we dive into this section, we will uncover why this technique is not just a passing trend but a vital tool for researchers.
Understanding the Need for Cloning
Cloning plays a pivotal role in genetic research, serving as the backbone of various applications ranging from gene expression to developing therapeutic proteins. Without cloning, it would be exceedingly challenging to isolate and study particular genes. In academic and commercial labs alike, cloning allows scientists to engineer organisms for specific traits, investigate genetic diseases, and even produce vaccines. The scalability and precision afforded by cloning mean that researchers can work with confidence, knowing they will achieve reliable results.
In this context, the adoption of efficient cloning techniques becomes of utmost importance. When the stakes are high—like in gene therapy or personalized medicine—having a reliable method can make all the difference. Topo cloning simplifies this intricate process, reducing potential delays and errors that can arise with traditional techniques. For students delving into molecular biology, understanding cloning is akin to learning how to read; it's fundamental.
Overview of Topo Cloning
Topo cloning is a method that utilizes the unique activity of topoisomerases—enzymes that manage DNA topology. This technique facilitates the insertion of DNA fragments into vectors more quickly and with increased efficiency. Essentially, the nature of topoisomerases allows for the creation of sticky ends on vectors, enabling direct ligation without the need for restriction enzymes or specialized adaptors.
One of the notable advantages of topo cloning is its speed. While traditional methods might drag on, topo cloning can yield results in mere hours, a crucial factor when time is of the essence in research projects. Moreover, the protocol generally provides a higher insert stability and lower background compared to other cloning techniques.
Because of these compelling benefits, topo cloning has found its way into many laboratories, solidifying its position as a go-to method for cloning tasks.
As we progress through this article, we will delve deeper into how topo cloning functions, what makes it distinct, and the best practices to ensure successful outcomes in your molecular biology endeavors.
Mechanism of Topo Cloning
The mechanism of topo cloning plays a pivotal role in the effectiveness and efficiency of this molecular biology technique. Understanding this mechanism is crucial for grasping how topo cloning simplifies the process of inserting DNA into vectors, ultimately facilitating various applications in genetic engineering and biotechnology. The focus here is not merely on the surface-level understanding but also on how the mechanisms laid out provide insights into optimizing the protocol for different laboratory contexts.
Enzymatic Basis of Topo Cloning
At the heart of topo cloning is the action of specific enzymes that facilitate the direct and seamless ligation of DNA inserts into vectors. This enzymatic process involves topoisomerases, which are proteins that manage the topological state of DNA. Unlike traditional ligation methods, which depend on generating compatible ends between vector and insert through restriction enzymes, topo cloning relies on the inherent properties of topoisomerases to promote ligation.
Initially, these enzymes introduce controlled breaks in the DNA backbone, allowing for the strands to rearrange freely.
- This leads to a unique mechanism where the insertion can occur without the need for blunt or sticky ends.
- Instead, the topoisomerase creates a temporary covalent link between the vector and the insert, thus expediting the entire process significantly.
This property not only increases the probability of successful ligation but also minimizes issues like incomplete reactions or unwanted by-products, which are common in traditional cloning techniques. The implication here is crystal clear: the enzymatic basis is what allows for a rapid, efficient, and versatile application of the cloning process.
Role of Topoisomerases
Topoisomerases are essentially the unsung heroes in the world of topo cloning. Their role goes beyond mere molecular cuts and joins; they bring precision and speed to the cloning process. Understanding their function reveals why topo cloning is so favored in contemporary genetic research.
The main types of topoisomerases, classified into Type I and Type II, operate by different mechanisms:
- Type I topoisomerases cleave one strand of the DNA, allowing the intact strand to pass through before resealing the break. This action effectively reduces supercoiling, facilitating the unwinding of DNA during replication and transcription.
- Type II topoisomerases, on the other hand, cut both strands of the DNA helix, allowing another double helix to pass through before rejoining the ends. This is particularly beneficial in settings that require substantial modifications to DNA structure.
"The precision of topoisomerases not only contributes to successful ligation but also minimizes insertion bias, a common pitfall in conventional cloning approaches."
"The precision of topoisomerases not only contributes to successful ligation but also minimizes insertion bias, a common pitfall in conventional cloning approaches."
As a direct consequence of these functionalities, researchers utilizing topo cloning are often met with higher transformation efficiency and less background noise compared to traditional methods. This makes the entire cloning endeavor not just faster, but also leaves less room for error, consequently enhancing the reliability of experimental outcomes.
In summary, both the enzymatic foundation laid by topoisomerases and their operational roles serve as the backbone of the topo cloning mechanism, making it a preferred choice among researchers aiming for precision and efficiency.
Step-by-Step Topo Cloning Protocol
The Step-by-Step Topo Cloning Protocol provides a clear and structured approach to manipulating genetic material, making it a significant focus in this guide. This section lays out the essentials and intricacies of the protocol for both novice and seasoned researchers. Understanding this step-by-step process is fundamental because it allows for precision in molecular cloning, ensuring successful results in various applications, from genetic engineering to protein analysis. The efficiency gained here can streamline research timelines and enhance experimental outcomes.
Preparation of Vector and Insert
Preparing the vector and the insert is the first and crucial step in the Topo Cloning Protocol. A vector acts as a vehicle to carry the DNA of interest into host cells, while the insert is the desired piece of DNA to be cloned.
To start, researchers should select a vector designed for Topo cloning, such as the pCR2.1 plasmid, which is often equipped with features that facilitate the insertion of DNA. For the insert, having the DNA fragment prepared with specific characteristics is key. The insert typically needs to have overhangs created by the topoisomerase enzyme, which facilitates the combination process.
Ensuring that both the vector and insert are of high quality is essential. Using a gel purification method can help here, as it removes unwanted fragments and allows for a pure sample. After purification, it is advisable to quantify the DNA using spectrophotometry, which can guide the following steps effectively. Achieving a good purity ratio (1.8 to 2.0) signals that the DNA is clean from contaminants and ready for the ligation step.
Ligation Process
Next comes the ligation process, where the vector and insert are combined. In Topo cloning, this process is facilitated by the action of topoisomerase enzymes that create a covalent bond between the vector and insert. This method ensures that the DNA ligation happens quickly, often within a mere 5 to 30 minutes.
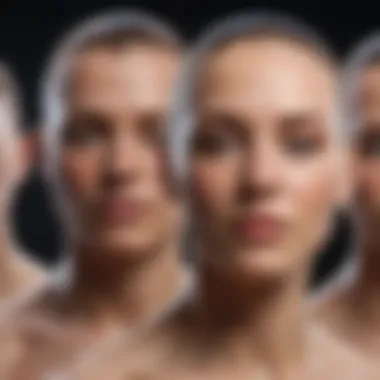
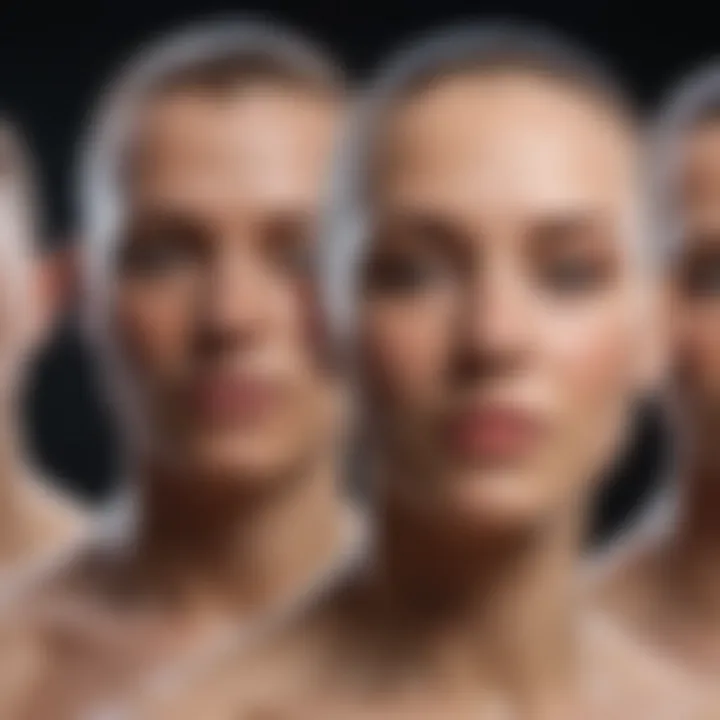
Researchers need to mix the prepared vector and insert in a ratio appropriate for the desired insert size. A common guideline suggests using a molar ratio of about 1:3 for vector to insert. The components are then incubated at room temperature. What sets Topo cloning apart is that the enzyme does much of the heavy lifting, making it a favored method for its speed and reliability.
Considerations during this phase include the potential need for a control reaction to compare results. This helps in identifying whether any transformations were successful or if background noise occurred during the experiment. Understanding the expected results of the successful ligation can help in troubleshooting later on.
Transformation into Competent Cells
The final step is the transformation into competent cells. This is the pivotal moment where the newly constructed DNA is introduced into host cells, typically E. coli for bacterial systems. The transformation process utilizes chemical methods or electroporation to allow the plasmid to penetrate the bacterial membrane.
After mixing the ligated DNA with the competent cells, a heat shock or electrical field is applied to facilitate uptake. Following transformation, the cells are typically incubated in a recovery medium to allow expression of the antibiotic resistance marker present on the vector, enhancing the survival of transformed cells.
It's also important to plate the cells onto agar plates containing the corresponding antibiotic. This helps in selecting only those cells that have successfully taken up the vector and insert. As the colonies grow, their number can reflect the efficiency of the transformation process.
Understanding these three components of the Topo Cloning Protocol not only provides a roadmap for academic study but also offers insights into practical applications. The proper execution of each step is critical for successful cloning, which can then power a range of molecular biology applications.
Key Takeaway: Preparation, ligation, and transformation are fundamental steps in Topo Cloning. Ensuring the quality of vector and insert, alongside proper protocols, can significantly impact experimental outcomes.
Key Takeaway: Preparation, ligation, and transformation are fundamental steps in Topo Cloning. Ensuring the quality of vector and insert, alongside proper protocols, can significantly impact experimental outcomes.
Applications of Topo Cloning
Topo cloning is like the Swiss army knife for molecular biology—it opens up a multitude of doors in the lab. This technique is not only convenient but also versatile, allowing researchers to tackle various challenges in genetic manipulation, expression studies, and more. With its precision and efficiency, topo cloning empowers scientists to make significant advances across several domains. Let’s dive into some specific applications.
Genetic Engineering
Genetic engineering stands as a cornerstone of modern biotechnology, enabling scientists to manipulate DNA with remarkable accuracy. Topo cloning plays a pivotal role here. By facilitating the insertion of specific DNA fragments into vectors, it allows for the creation of recombinant DNA, which is essential for producing genetically modified organisms (GMOs).
Benefits include:
- Precision: Topo cloning provides a reliable means to incorporate desired sequences with minimal unintended changes, which is crucial in maintaining gene integrity.
- Flexibility: Researchers can easily utilize a range of vectors tailored to specific functions, enhancing their ability to develop unique strains or modified organisms.
- Rapid Outcomes: The speed of topo cloning translates into quicker results compared to traditional techniques, allowing for faster experimentation and development cycles.
Moreover, practices like gene editing, such as CRISPR-Cas9, often rely on swift and accurate cloning methods like topo cloning to prepare for more complex manipulations.
Protein Expression Studies
Protein expression is another critical application where topo cloning shines. In order to understand how proteins function, it’s essential to express them in host systems, and topo cloning streamlines this process. By cloning genes of interest into expression vectors, researchers can produce proteins for a wide variety of applications ranging from therapeutic use to industrial enzymes.
Key considerations include:
- Choice of Host: The ability to select suitable hosts such as E. coli or yeast is facilitated by topo cloning, depending on the protein's characteristics and requirements.
- Tagging: Researchers often attach tags to proteins via cloning, simplifying purification processes and allowing for better tracking during experiments.
- Functional Analysis: Amplifying and expressing proteins helps in elucidating their roles in cellular processes, paving the way for drug discovery and biomarker development.
In essence, topo cloning reduces the hurdles that often accompany protein production and analysis, allowing for breakthroughs in understanding biological mechanisms.
Mutational Analysis
Understanding mutations is key to exploring genetic variation and its implications. Topo cloning serves as an invaluable tool in mutational analysis, as it simplifies the introduction of specific changes into a DNA sequence. This is essential for studying gene function and the consequences of genetic alterations.
Important aspects include:
- Site-Directed Mutagenesis: By enabling precise modifications, researchers can comprehensively investigate the role and impact of specific nucleotides or amino acids in genes and proteins.
- Functional Studies: Introduced mutations can lead to alterations in protein function, offering insights into pathways involved in diseases, such as cancer.
- High Throughput Capabilities: When paired with techniques like next-generation sequencing, topo cloning allows for the simultaneous analysis of multiple variants, rapidly enriching data for evolutionary studies.
Thus, topo cloning does not merely facilitate experiments; it transforms them into avenues of discovery that could lead to innovative solutions in genetics and beyond.
Advantages of Topo Cloning
Understanding the advantages of topo cloning is paramount, especially in a landscape where precision and efficiency are crucial for molecular biology research. This technique presents several key benefits that can significantly transform workflow within laboratories, paving the way for groundbreaking discoveries.
Speed and Efficiency
One of the standout features of topo cloning is its impressive speed and efficiency. Traditional cloning methods often drag on, involving multiple steps that can take days or even weeks to yield results. The beauty of topo cloning lies in its ability to streamline the process, condensing it into mere hours. The mechanism by which topo cloning allows for this quick turnaround revolves around the unique properties of topoisomerases, enzymes that facilitate the insertion of DNA fragments into vectors without the need for extensive ligation procedures.
For instance, consider a researcher looking to clone a specific gene. With topo cloning, they can prepare their vector and insert concurrently, often eliminating many of the preparatory complexities typical in conventional cloning methods. This not only saves time but also minimizes the risk of contamination that can arise from prolonged sample handling.
Additionally, the user-friendly protocols involved, whether utilizing commercial kits or lab-prepared components, enable researchers to bypass some of the more labor-intensive aspects of molecular cloning.
Reduced Background
Another significant advantage of topo cloning is the reduced background noise in comparison to traditional methods. Background colonies—those unwanted bacterial colonies on selection plates—can often muddy results, leaving researchers questioning the integrity of their findings. With topo cloning, the targeted nature of the method inherently minimizes such occurrences.
This reduction can largely be attributed to the high specificity of how the topoisomerase works in catalyzing the reaction between vector and insert. The selective nature of the process permits only those DNA fragments that match the vector design to be successfully ligated, thereby decreasing the chances of non-specific insertions.
"By employing topo cloning, researchers can be more confident in the results of their experiments, knowing that the data they gather is less likely to be compromised by extraneous variables stemming from unwanted colonies."
Moreover, this specificity can also lead to fewer wasted resources on unnecessary follow-up experiments to sift through unhelpful data.
To summarize:
- Speed and efficiency fundamentally enhance research timelines.
- A more predictable outcome translates to higher reproducibility and reliability in experimental results.
- Reduced background noise means cleaner data and reduced variability, a key factor in advanced research methodologies.
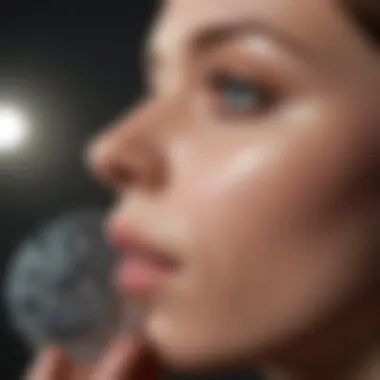
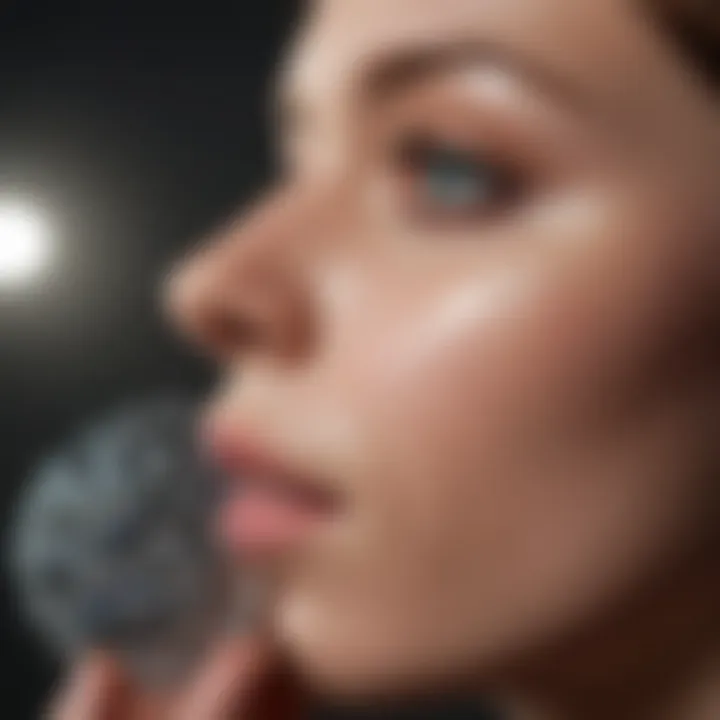
Incorporating topo cloning into research practices not only simplifies the overall process but also equips researchers with powerful tools to pursue innovative avenues in molecular biology.
Limitations and Challenges
Understanding the limitations and challenges inherent in the topo cloning protocol is essential for researchers aiming to maximize its potential. While this technique is heralded for its efficiency and speed compared to traditional cloning methods, it is not without its drawbacks. Recognizing these limitations is invaluable for troubleshooting and refining research strategies, ultimately ensuring better outcomes in experiments.
One of the primary challenges faced by scientists is insertion bias. This phenomenon occurs when the enzyme involved in the cloning process favors certain sequences or orientations for insertion, often leading to an unintended skew in the types of inserts that are successfully integrated into the vector. Consequently, this may result in underrepresentation or overrepresentation of specific genetic elements, thereby complicating the interpretation of experimental results. It’s like trying to fit a square peg into a round hole – the peg may fit, but can you be sure it’s the right one?
Insertion Bias
Insertion bias can manifest in several ways and presents unique hurdles. For instance, topo cloning often preferentially inserts fragments with certain sequence traits. This can skew the data, making it difficult to draw accurate conclusions from the experiment. The reliance on the topoisomerase mechanism, which catalyzes the formation of covalent bonds with DNA, means that only sequences recognizing the enzyme's specific affinity might be cloned efficiently. Researchers could mitigate these pitfalls by designing their insert sequences carefully, aiming for the diversity in the lost regions. However, this requires a deeper understanding of the enzyme’s behavior, sometimes leading to painstaking trial-and-error processes.
It’s critical to consider these biases in experimental designs to ensure reliable results. Acquiring a range of insert variants or maintaining a careful selection process may help balance potential skewing. Moreover, enhancing protocols with controls or alternative cloning strategies could also minimize the impacts of insertion bias, allowing for more comprehensive analyses.
Vector Compatibility Issues
Another notable challenge lies within vector compatibility issues. Not all vectors are created equal, and their interactions can sometimes lead to complications. The effectiveness of a topo cloning experiment hinges not only on the insert but on how well the vector accommodates these inserts. Variables such as insert length, the presence of secondary structures, and the vector's own characteristics can all affect compatibility.
When a vector does not match well with an insert, the resultant transformation can yield unexpected outcomes – think of it as mismatched shoes; they might fit on your feet, but they won't help you walk effectively. This can plummet transformation efficiency and lead to spikes in background colonies, which is frustrating for any researcher looking for precision.
To navigate these complications, scientists should carefully choose vectors that are well-established for their specific inserts. Exploring options like using dual-promoter vectors or ones with adjustable selection markers can provide flexibility. Additionally, running thorough compatibility tests might save researchers precious time and resources during the subsequent stages of their projects.
In summary, while topo cloning represents a notable advancement in molecular biology, its limitations and challenges require thoughtful consideration and strategic approaches to overcome. Being aware of issues like insertion bias and vector compatibility can inform better experimental design, leading to more reliable findings and fostering more innovative research moving forward.
"It’s not the challenges we face that define us, but how we respond to them."
"It’s not the challenges we face that define us, but how we respond to them."
By navigating these challenges judiciously, researchers can harness the full potential of topo cloning while minimizing its pitfalls.
Modifications to the Standard Protocol
When working with the topo cloning technique, researchers often encounter scenarios that necessitate tweaks or modifications to the standard protocols. These adjustments can greatly enhance the efficiency and specificity of the cloning process, making them an important focus in any comprehensive guide on topo cloning. Understanding these modifications is essential as they pave the way for more successful experimental outcomes and deeper insights into genetic manipulation.
Use of Alternative Enzymes
The enzymatic component of topo cloning is critical, as it directly influences the efficiency of the process. Traditional topoisomerases, specifically Type I, are commonly employed in standard protocols. However, researchers may opt for alternative enzymes based on their cloning objectives or specific experimental conditions.
For instance, using a higher-fidelity enzyme can minimize the likelihood of generating mutations during the cloning process. This is particularly imperative when the end goal involves expressing proteins that require exact amino acid sequences to maintain their functions. On the flip side, some may choose to adopt thermophilic enzymes for cloning at elevated temperatures which often leads to better stability and activity.
"As research progresses, adapting methodologies with different enzymes can yield superior results compared to rigidly sticking to traditional approaches."
"As research progresses, adapting methodologies with different enzymes can yield superior results compared to rigidly sticking to traditional approaches."
The selection of these alternative enzymes also considers factors like cost, availability, and specific activity. Thus, one must weigh these components carefully. A little investigation into supporting literature or current trends in microbiological research can guide these decisions effectively.
Enhanced Vectors
Another significant modification to standard protocols involves the use of enhanced vectors. Vectors are the vehicles that carry the genetic material to be cloned. Using conventional vectors might often suffice, but the introduction of improved vectors can lead to markedly better results.
Enhanced vectors often come with built-in features that facilitate easier screening of clones. For instance, vectors might incorporate multiple cloning sites (MCS) which allows for the insertion of various DNA fragments with greater flexibility. Additionally, some modern vectors are designed to include selectable markers that ensure only successfully transformed cells survive, thereby streamlining the selection process.
Moreover, certain vectors are equipped with promoters that can significantly increase the yields of the desired protein, which is particularly advantageous in protein expression studies. Researchers might find themselves grappling with the realities of vector stability within different host organisms, thus experimenting with engineered versions can offer the precision needed for their specific application.
In essence, the modifications in both enzymes and vectors serve as powerful tools within the topo cloning framework. By choosing alternatives that align closely with specific project goals, researchers enhance their chances of achieving desired outcomes. Exploring these options not only builds a deeper understanding of the cloning process but also refines the quality of the research being conducted.
Troubleshooting Common Issues
In any scientific endeavor, especially within the realm of molecular biology, the pathway isn’t always smooth; bumps and hurdles often crop up along the way. This section on troubleshooting common issues surrounding topo cloning aims to arm researchers with the knowledge needed to effectively address these challenges. Recognizing and understanding the pitfalls can not only save time but also enhance the reliability of the results. The significance of tackling these common issues effectively cannot be understated, as it fosters both accuracy and efficiency in experiments.
Low Transformation Efficiency
Low transformation efficiency can often be a nagging issue that researchers encounter when working with topo cloning protocols. Essentially, transformation efficiency refers to the number of bacterial cells that successfully take up the plasmid DNA, a measure fundamental to the success of cloning endeavors. If the efficiency is lower than anticipated, it might feel like trying to catch smoke with your bare hands.
There could be several reasons for low transformation efficiency:
- Quality of DNA: If the DNA is degraded or poorly prepared, the chances of successful transformation diminish significantly.
- Competent Cells: The competency of the bacterial strain used is crucial. Over time, competency can decrease, especially if cells have been mishandled or improperly stored.
- Ligation Conditions: Suboptimal ligation reactions can produce a mixture that is less favorable for transformation.
Mitigating this issue typically involves several strategies:
- Confirm the integrity of the DNA by running it through a gel electrophoresis; purify it if necessary.
- Ensure that competent cells are freshly prepared or bought from reliable sources.
- Optimize the ligation reactions, perhaps by tweaking enzyme concentrations or reaction times.
Ultimately, a systematic approach to diagnosis can reveal the underlying causes of low transformation efficiency, leading to better experimental outcomes.
Background Colonies
Background colonies, those pesky little structures that pop up on agar plates seemingly out of nowhere, can drastically skew the accuracy of your results in topo cloning. These colonies arise due to non-specific amplification or the presence of uninserted vector DNA, and can be a real annoyance, making it hard to discern the successful colonies containing the insert from the false positives.
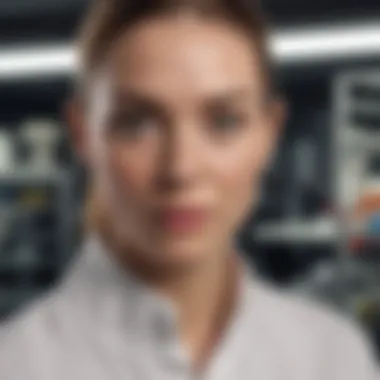
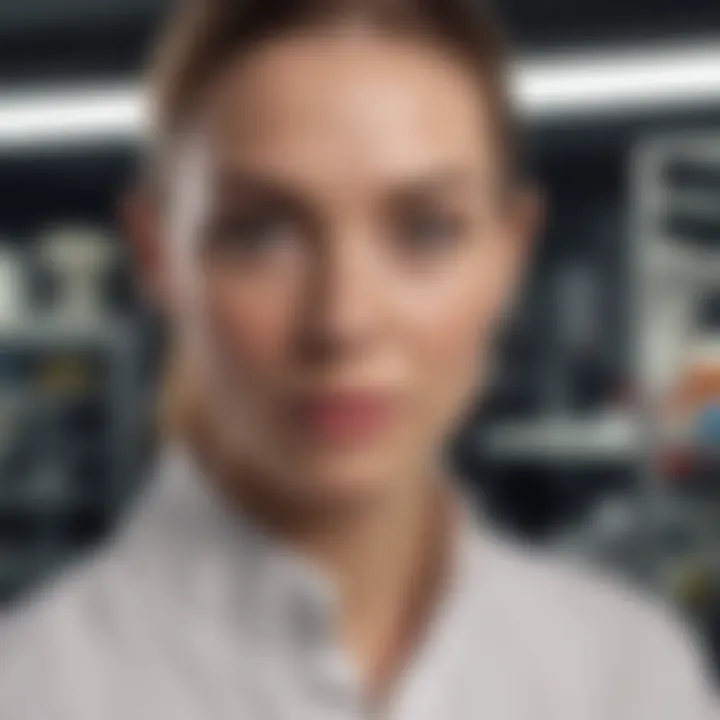
To deal with background colonies effectively, here are tailored approaches:
- Digesting Vector DNA: Performing a double digest before ligation can significantly reduce the chances of background colonies by ensuring that only correctly ligated insert vector combinations can survive.
- Use of Selective Markers: Introducing selectable markers can help ensure that only the colonies carrying the insert can grow under specific conditions, making it easier to distinguish them from unwanted colonies.
- Optimizing Transformation Protocols: Sometimes, simply optimizing the heat-shock conditions or recovery periods can decrease the background, leading to cleaner results.
"Reducing background colonies makes the hunt for successful clones much less like going on a wild goose chase."
"Reducing background colonies makes the hunt for successful clones much less like going on a wild goose chase."
In essence, dealing with background colonies requires both a proactive approach and creative thinking. This objective calls for a keen eye, as well as a willingness to tweak and adjust methods to suit the particular experiment.
Through addressing these common issues with meticulous attention and resourceful strategies, researchers can ultimately enhance the success rates of their cloning projects and bring clarity to their findings.
Comparative Analysis with Other Cloning Techniques
In the world of molecular biology, cloning techniques are essential tools that researchers employ to manipulate DNA for various applications. A comparative analysis not only sheds light on the distinctive features of these techniques but also helps highlight their strengths and weaknesses. The importance of this comparison cannot be overstated; it provides insights needed for researchers to select the most suitable method based on their specific requirements.
An in-depth look at various cloning strategies offers a clearer understanding of where topo cloning fits into the larger picture. These comparisons can guide scientists in making informed choices about which method aligns best with their experimental goals.
Traditional Restriction Cloning
Traditional restriction cloning is perhaps the most conventional approach. It hinges on the use of restriction enzymes that cut DNA at specific sequences. This allows researchers to splice together fragments of DNA with high precision.
**Key Points:
- Enzyme dependence:** This method relies heavily on the availability and specificity of restriction enzymes. Researchers might find certain enzymes that cut too infrequently or not at all, complicating the process.
- Directional cloning: The standard protocol often requires careful planning to ensure that insertions happen in the desired orientation. This can lead to time-consuming steps.
Due to its roots in the early days of molecular biology, traditional restriction cloning is well-understood. However, several drawbacks exist; for instance, the possibility of star activity—where enzymes cut at unintended sites—can hinder outcomes.
Gibson Assembly
Gibson assembly is a more recent and increasingly popular technique. This method is noted for its ability to join multiple DNA fragments in a single, seamless reaction without the need for restriction enzymes. Instead, it employs a combination of Exonuclease, DNA Polymerase, and DNA Ligase.
Advantages of Gibson Assembly:
- Flexibility: One of the hallmarks of this technique is the ability to construct large fragments of DNA, making it advantageous for building entire genes or operons.
- Single-tube reaction: The assembling of fragments in a single reaction streamlines the workflow, reducing the risk of contamination and saving time.
- Homology-based joining: Utilizing overlapping regions on each fragment enhances precision, potentially leading to higher success rates in building clones.
However, it also comes with its own set of challenges. For instance, designing the overlapping sequences requires meticulous attention to detail, and some laboratories might find variations in efficiency depending on the DNA sequences utilized.
By understanding different cloning methodologies, researchers can leverage the unique advantages of each to optimize their experimental outcomes.
By understanding different cloning methodologies, researchers can leverage the unique advantages of each to optimize their experimental outcomes.
In summary, the comparative analysis with traditional restriction cloning and Gibson assembly reveals significant diversity within cloning techniques. Topo cloning holds its ground with unique advantages in speed and simplicity, making it a strong contender in the toolbox of genetic engineering. Understanding how it stacks up against other methodologies provides critical insight for researchers aiming to navigate the complexities of molecular cloning.
Future Perspectives in Topo Cloning
The realm of topo cloning is continuously evolving, and so are the possibilities it presents to molecular biology. As research methodologies grow in sophistication, assessing the future of topo cloning becomes vital. This importance lies not only in assessing what works but also what can be modified, integrated, or completely innovated upon. The advancements emerging today pave the pathway for optimizing cloning techniques and, therefore, enhancing research outcomes.
Emerging Technologies
Emerging technologies are driving the transformation landscape for topo cloning. As scientists leverage advancements in various fields, the tools and methods we use are bound to change. Here are several notable areas where we can expect substantial progress:
- CRISPR-Cas9 Integration: The fusion of topo cloning with CRISPR technology has taken the genetic editing field by storm. By using topo cloning as a precursor step, researchers can make accurate insertions or modifications within the genome, making the process faster and more efficient.
- Nanotechnology Applications: Innovations within nanotechnology are offering new materials that enhance vector and insert stability. This can lead to higher transformation efficiencies and lower background noise during experiments, which is crucial for achieving reliable results.
- Automated Cloning Platforms: Robotics and machine learning are on the horizon for techniques like topo cloning. Automating the steps can minimize human error, thereby increasing consistency across experiments. This could replace tedious manual processes with efficient, streamlined workflows.
The implications for these technologies are numerous. If adopted, they can markedly improve the precision and reliability of molecular cloning experiments while making the process more accessible for researchers.
Integration with Other Techniques
The integration of topo cloning with other cloning techniques paints a promising future for molecular biology. This fusion may not only enhance the efficiency but could also lead to novel methodologies that outperform conventional ones. Some valuable integrations include:
- Linking with Traditional Restriction Enzyme Cloning: The combination allows for a more versatile approach, enabling researchers to use topoisomerases' speed alongside the specificity of restriction enzymes. This dual approach can cater to a wider range of applications.
- Combining with Gibson Assembly: By leveraging both topo cloning and Gibson assembly, one could utilize the advantages of seamless cloning while maintaining the rapid insertion capabilities of topo cloning. This could be particularly useful in constructing large or complex plasmids.
- Incorporating Synthetic Biology Techniques: With the rise of synthetic biology, merging topo cloning with synthetic DNA assembly presents a unique opportunity. This can aid in designing whole pathways or larger constructs in a more efficient manner, turning complex biological functions into manageable tasks.
Through these varied integrations, topo cloning can adapt to changing scientific landscapes, addressing the challenges and needs of modern research. Together, these emerging technologies and integrations paint a hopeful picture of where topo cloning can lead us in the years to come.
End
The conclusion section serves as a pivotal wrap-up for this comprehensive discussion on topo cloning. Wrapping up the various insights shared, it stands crucial not just to summarize the contents but to drive home the lasting relevance of the topo cloning technique in contemporary molecular biology research. Each component of the protocol, its application, and the surrounding methodologies play a vital role in fostering an understanding of genetic manipulation.
Summary of Key Points
To reiterate, several key elements have been highlighted throughout the article:
- Need for Cloning: Understanding the driving reasons behind the necessity of cloning, particularly in genetic research, can guide future innovations.
- Mechanism: The mechanistic foundations of topo cloning set it apart from other methods, rooted in its specific enzymatic activities involving topoisomerases.
- Applications: The versatility of topo cloning stretches across various domains such as genetic engineering and protein expression—a testament to its utility in the lab.
- Advantages and Limitations: Despite its benefits including speed and reduced background issues, it is imperative to acknowledge the limitations, notably insertion bias and compatibility challenges.
- Troubleshooting: Having a troubleshooting framework enhances the protocol's reliability, enabling researchers to effectively navigate common hiccups encountered during experimentation.
- Future Perspectives: Emerging technologies and their integration with traditional methodologies will ensure that topo cloning remains at the forefront of genetic and biotechnological advancements.
Final Thoughts on Topo Cloning
In closing, the significance of topo cloning goes well beyond mere lab procedures; it represents an evolving landscape within the field of genetic manipulation. The adaptability of this protocol speaks volumes about its place in the toolkit of researchers aiming for precision in their work. As molecular biology continues to advance, the insights gathered from this exploration serve as a cornerstone for future studies.
Ultimately, a deeper comprehension of methods like topo cloning paves the way for groundbreaking discoveries in genetics and biotechnology. Understanding its nuances not only fosters more effective research but also ignites curiosity and innovation within the scientific community.
"In science, a technique's value lies in its ability to uncover truths that were previously hidden."
"In science, a technique's value lies in its ability to uncover truths that were previously hidden."
Whether one is a student just embarking on their educational journey or a seasoned professional, the insights from this guide are meant to enrich one’s understanding of topology in molecular cloning. As we stride forward into new territories of genetic research, topological considerations will remain central to our discoveries.