Understanding RNA: Functions and Implications
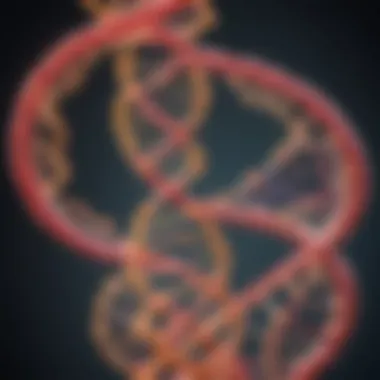
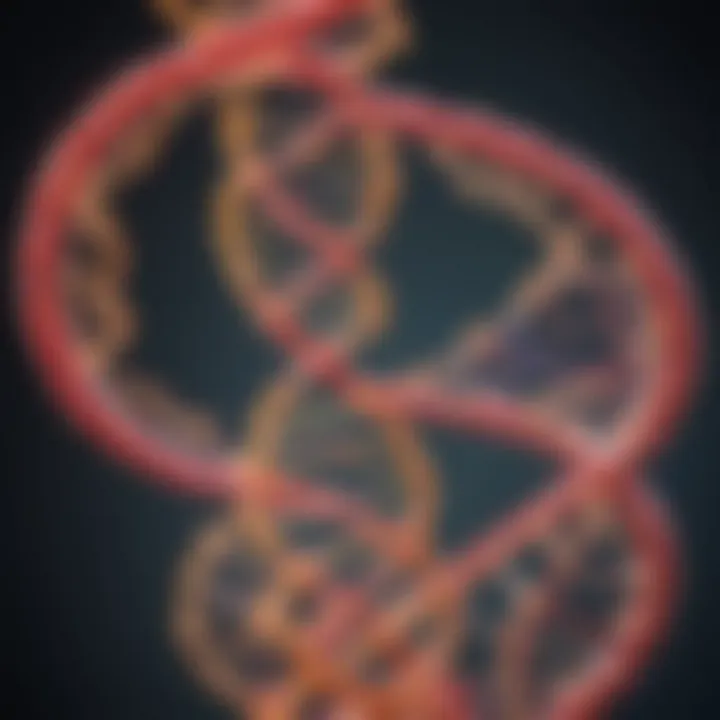
Intro
Ribonucleic acid, commonly known as RNA, is a vital molecule in biological systems. It plays a fundamental role in various cellular processes, especially in genetics and protein synthesis. An understanding of RNA encompasses its structure, types, and functions. This guide aims to provide insights into the intricacies of RNA and its implications in modern science and medicine.
Key Concepts
Definition of the Main Idea
RNA is a nucleic acid that consists of long chains of ribonucleotides. Each nucleotide is made up of three components: a ribose sugar, a phosphate group, and a nitrogenous base. Unlike deoxyribonucleic acid (DNA), RNA is typically single-stranded, which allows it to perform a variety of functions in the cell. It exists in several forms, including messenger RNA (mRNA), transfer RNA (tRNA), and ribosomal RNA (rRNA), each serving a unique purpose in the flow of genetic information.
Overview of Scientific Principles
RNA plays a crucial role in translating genetic information from DNA into proteins. This process can be divided into three main steps: transcription, translation, and post-translational modification. During transcription, mRNA is synthesized from a DNA template. This mRNA then carries genetic information to ribosomes, where proteins are assembled in a process called translation. tRNA facilitates the incorporation of the correct amino acids into the growing polypeptide chain.
It is essential to note that RNA is not just a messenger between DNA and proteins but also functions in regulation and catalysis. Some RNA molecules, known as ribozymes, have enzymatic activity, suggesting that RNA may have played a critical role in the early evolution of life.
RNA is an essential player in the genetic expression and regulation, shaping the functioning of all living organisms.
RNA is an essential player in the genetic expression and regulation, shaping the functioning of all living organisms.
Current Research Trends
Recent Studies and Findings
Current research in RNA focuses on its diverse roles in cellular processes and disease mechanisms. Studies have shown that non-coding RNAs, which do not encode proteins, are crucial for regulating gene expression and managing cellular functions. The understanding of microRNAs and long non-coding RNAs is expanding, revealing their implications in development, cancer, and various diseases.
Significant Breakthroughs in the Field
Significant breakthroughs include advancements in RNA technology, such as RNA interference (RNAi) and CRISPR-based techniques. These innovations offer revolutionary strategies for gene editing and therapeutic interventions. Researchers have begun to explore RNA as a target for drug discovery, utilizing its unique properties to develop molecular therapies.
Defining RNA
Ribonucleic acid (RNA) is a central molecule in the understanding of molecular biology and genetics. Defining RNA allows for addressing its multiple roles in living organisms. This section will explore its basic definition and how it uniquely contributes to cellular functions, distinguishing it from other crucial nucleic acids.
What is Ribonucleic Acid?
Ribonucleic acid, commonly known as RNA, is a polymer made up of nucleotide monomers. Each nucleotide consists of a ribose sugar, a phosphate group, and a nitrogenous base. RNA comes in various forms with distinct functions, most notably messenger RNA (mRNA), transfer RNA (tRNA), and ribosomal RNA (rRNA). These types of RNA are integral to the processes of transcription and translation, which are fundamental to gene expression. In contrast to DNA, which serves as the long-term archive of genetic information, RNA plays a more immediate role in protein synthesis and regulation of gene activity.
Comparison with Other Nucleic Acids
DNA vs RNA
The main aspect that distinguishes DNA from RNA is their structure and function. DNA is typically double-stranded and forms a helical shape, while RNA is usually single-stranded. This difference is not merely structural; it has significant implications for their roles in the cell. DNA's stability is crucial for long-term genetic storage, whereas RNA's more versatile nature allows it to be synthesized on demand, allowing cells to respond rapidly to changing conditions. This property makes RNA a beneficial focus in studies related to gene expression and regulation.
Key structural differences
There are critical structural differences between RNA and DNA. While DNA contains deoxyribose as its sugar component, RNA contains ribose. The presence of an additional hydroxyl group in ribose makes RNA more reactive and less stable than DNA. This instability can be advantageous in roles where quick synthesis and degradation of genetic information is needed, such as in gene regulation and metabolic pathways. Moreover, the nitrogenous bases differ; RNA utilizes uracil in place of thymine found in DNA. This substitution influences how RNA molecules function within various biological processes, offering capabilities that DNA cannot.
"Understanding the key differences between RNA and DNA is essential for grasping the intricate mechanisms of genetic expression and regulation."
"Understanding the key differences between RNA and DNA is essential for grasping the intricate mechanisms of genetic expression and regulation."
In summary, defining RNA is essential for recognizing its versatile roles in the cell and its differences from DNA. Such distinctions underscore the significance of RNA in biotechnology and medical research, making it a crucial area of study.
Structure of RNA
RNA, or ribonucleic acid, has a fundamental role in various biological processes. Understanding its structure is crucial for grasping how RNA functions in genetics and overall cellular activities. The structure of RNA influences its stability, functionality, and interactions with other biomolecules.
Nucleotide Composition
Components of RNA nucleotides
The building blocks of RNA are comprised of nucleotides. Each nucleotide consists of three main components: a ribose sugar, a phosphate group, and a nitrogenous base. The ribose sugar is a five-carbon sugar that distinguishes RNA from DNA, which contains deoxyribose. This subtle difference affects the stability of the molecule since RNA is generally more prone to hydrolysis than DNA. The phosphate group provides the negative charge that aids in the interaction with proteins and other molecules, enhancing its biological role.
The unique structure of RNA nucleotides is vital in defining the properties of RNA. The presence of a hydroxyl group at the 2' position in the ribose sugar is a critical characteristic, as it allows for more flexibility and a varied conformation in RNA structures compared to DNA. This flexibility is beneficial, as it allows RNA to adopt different shapes necessary for its diverse functions in the cell.
Types of nitrogenous bases
RNA can contain four different nitrogenous bases: adenine, uracil, cytosine, and guanine. Unlike DNA, which contains thymine, RNA uses uracil instead, which pairs with adenine during base pairing. This change in composition gives RNA its unique properties. The presence of uracil is not only a defining feature but also has implications for RNA's interactions and stability.
The types of bases contribute to the molecule's overall stability and functionality. The ability of these bases to form hydrogen bonds determines how RNA folds and interacts within the cell. The unique pairing interactions of RNA bases facilitate crucial processes like transcription and translation, making them essential for gene expression.
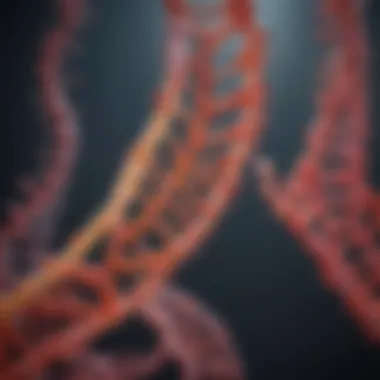
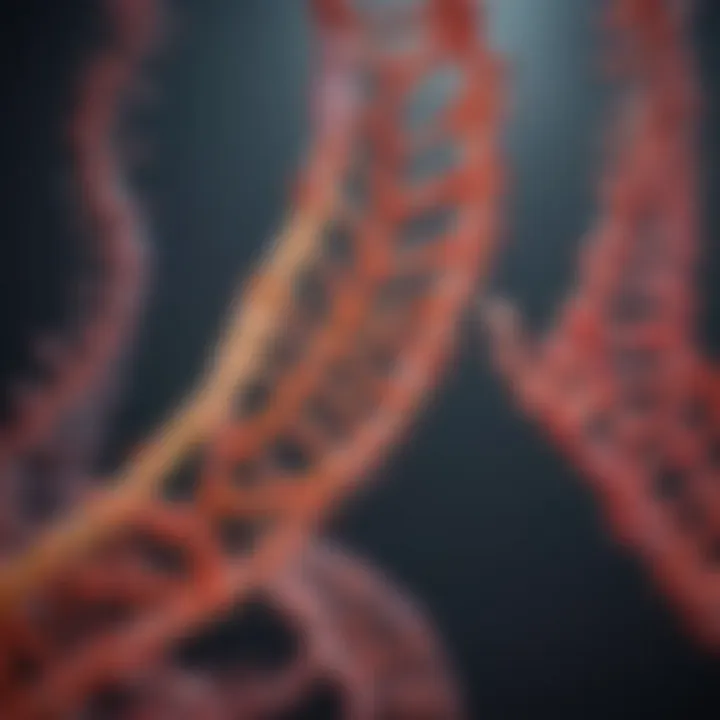
Types of RNA Structures
Single-stranded RNA
Single-stranded RNA (ssRNA) is the most common form of RNA found in cells. This structure allows for a variety of secondary formations, such as loops and hairpins. These configurations are significant for function, as they can influence the ability of RNA to pair with proteins and other nucleic acids.
The flexibility of single-stranded RNA is a key characteristic, allowing it to perform a variety of roles. This adaptability makes ssRNA beneficial in instances such as coding for proteins or regulating gene expression. Furthermore, the unique structure of ssRNA can lead to specific interactions that are essential for cellular processes. However, its single-stranded nature makes it more susceptible to degradation compared to double-stranded forms.
Double-stranded RNA
Double-stranded RNA (dsRNA) differs in structure, presenting a helical format similar to that of DNA. This arrangement adds a layer of stability due to the base pairing between strands. dsRNA is frequently found in certain viruses and plays important roles in the cell’s response to viral infections.
The key advantage of double-stranded RNA is its stability, making it less likely to be degraded compared to single-stranded forms. The unique feature of dsRNA allows for effective interaction with various proteins, including those involved in RNA interference mechanisms. However, it can also pose challenges when considering how the immune system responds to such structures, as it may trigger unwanted inflammatory responses.
In summary, the structure of RNA, including its nucleotide composition and the nature of its single-stranded or double-stranded form, is critical for understanding its diverse functions. The variations in RNA structure enable it to participate actively in cellular processes, gene expression, and regulatory mechanisms.
Types of RNA
The study of RNA encompasses several forms, each with unique functions vital for cellular operations. Understanding the different types of RNA is essential as it provides insight into the mechanisms of genetic expression, regulation, and the broader implications for biotechnology and medicine.
Messenger RNA (mRNA)
Function in protein synthesis
Messenger RNA plays a crucial role in protein synthesis. It serves as the template for translating genetic information into proteins, which are essential for various biological functions. mRNA's ability to carry specific coding sequences allows for the synthesis of proteins by ribosomes. This characteristic is significant because proteins are responsible for performing most life functions, thus highlighting mRNA's importance in cellular biology.
A distinct feature of mRNA is its sequence structure, which corresponds directly to the gene from which it is transcribed. Its linear arrangement allows for the precise translation of amino acids during protein assembly. This accuracy facilitates the creation of functional proteins necessary for health and survival. However, mRNA is also relatively unstable and has a short lifespan, which can be a disadvantage in certain contexts.
Transcription process
The transcription process is the initial step in gene expression, where DNA is copied into messenger RNA. This process is critical as it sets the stage for subsequent translation into proteins. Transcription involves various enzymes, notably RNA polymerase, which synthesizes mRNA by complementary base pairing with the DNA template.
A key characteristic of this process is its highly regulated nature, ensuring that specific genes are expressed at the right time and place. This regulatory aspect contributes to the diversity of proteins produced in an organism. The unique feature of transcription lies in its ability to produce multiple mRNA variants from a single gene, allowing for alternative splicing. While this flexibility is beneficial, it also adds complexity to the regulation of gene expression.
Transfer RNA (tRNA)
Role in translation
Transfer RNA is equally important in the protein synthesis process. tRNA's primary role is to transport specific amino acids to ribosomes, where they are assembled into polypeptides. This function directly ties tRNA to the translation stage of protein synthesis. One significant aspect of tRNA is its structure, which includes an anticodon that pairs with the corresponding codon on mRNA during translation. This characteristic ensures that amino acids are added in the correct order.
The unique feature of tRNA is its ability to recognize specific codons through its anticodon, which facilitates the accurate incorporation of amino acids. Though highly effective, tRNA relies heavily on the accuracy of the codon-anticodon interactions, where any mismatch could lead to protein malfunction.
Anticodon recognition
Anticodon recognition is a critical element of tRNA functionality. Each tRNA molecule has a specific anticodon sequence, allowing it to bind to the corresponding codon on the mRNA strand during translation. This process is essential for ensuring that the correct amino acid is brought to the growing peptide chain. The characteristic nature of anticodon recognition makes it a fundamental aspect of the protein synthesis process.
What sets this recognition apart is how it adds specificity to the translation process. Each tRNA is linked to a specific amino acid, and its ability to accurately recognize codons ensures that proteins are synthesized with high fidelity. While this feature is advantageous for protein synthesis, it also presents challenges, particularly with mutations in mRNA that could alter codon sequences.
Ribosomal RNA (rRNA)
Structure of ribosomes
Ribosomal RNA constitutes a key structural component of ribosomes. Ribosomes are the cellular machinery responsible for translating mRNA into proteins. The architecture of ribosomes includes both rRNA and proteins, but it is the rRNA that primarily provides the structural framework necessary for ribosomal function.
This relationship between rRNA and ribosome structure is significant as it plays a role in stabilizing the ribosomal complex during translation. The unique feature of rRNA is its catalytic function, where it not only serves as a scaffold but also facilitates peptide bonding between amino acids, demonstrating an essential aspect of the translation mechanism. While rRNA is abundant and variations exist, certain modifications can impact ribosomal function and potentially lead to disease.
Role in protein synthesis
The role of ribosomal RNA in protein synthesis extends beyond mere structure. rRNA also plays a direct role in the catalytic action of the ribosome during translation. By forming the peptidyl transferase center, rRNA catalyzes the formation of peptide bonds between amino acids. This highlights the functional importance of rRNA within the synthesis machinery.
The characteristic of rRNA's involvement in translation makes it vital for life. Its unique ability to catalyze reactions makes rRNA one of the few known RNA molecules with enzymatic activity. However, its reliance on precise interactions with mRNA and tRNA means that errors in rRNA can critically affect protein production, affecting overall cell function.
Non-coding RNAs
Examples of non-coding RNAs
Non-coding RNAs are RNA molecules that do not translate into proteins but have critical regulatory functions in cells. One prominent group includes microRNAs, which are involved in gene regulation by targeting mRNAs for degradation or inhibition. Another example is long non-coding RNAs (lncRNAs), which play roles in chromatin remodeling, gene transcription, and RNA processing.
The significance of non-coding RNAs lies in their diverse functional roles beyond mere transcription. These molecules provide essential methods for controlling gene expression, illustrating the complexity of genetic regulation. A particular advantage of these non-coding RNAs is their ability to regulate multiple mRNAs simultaneously, thus affecting a broader array of cellular pathways.
Functions and significance
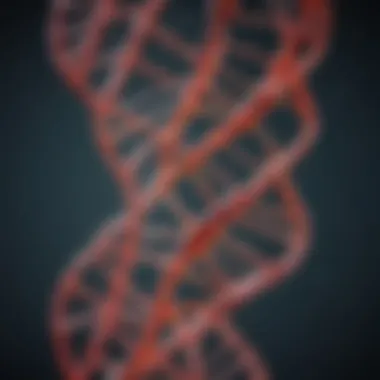
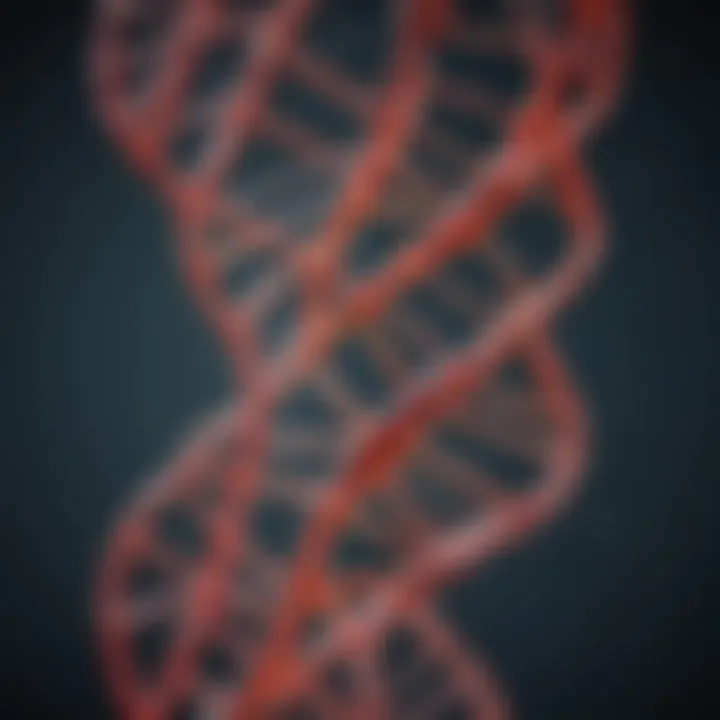
The functions of non-coding RNAs emphasize their importance in various biological processes. They are involved in regulating gene expression at multiple levels, including transcription and post-transcriptional modification. This highlights a characteristic of non-coding RNAs that identifies them as essential players in cellular regulation.
The unique aspect of non-coding RNAs is their ability to influence gene expression networks, thereby impacting overall cellular behavior. Their regulatory roles can confer advantages by allowing organisms to fine-tune responses to environmental changes. However, misregulation of non-coding RNAs has been implicated in several diseases, posing challenges for understanding their precise mechanisms and potential therapeutic targets.
Understanding the diverse types of RNA allows for deeper insights into biological processes and implications for treating diseases. RNA continues to be a focus of research, as its roles extend into numerous critical areas of science.
Functions of RNA
The functions of RNA are pivotal in molecular biology, influencing gene expression and regulatory mechanisms. This section investigates how RNA transcends its structural role, acting as an essential player in various biological processes. Understanding RNA's functions can help elucidate its significance in genetic manipulation and therapeutic applications.
Role in Gene Expression
Gene expression is a multifaceted process where RNA plays a central role, acting as a bridge between DNA and protein production. This process includes two primary stages: transcription and translation, both essential for the proper expression of genes.
Transcription
Transcription is the initial step in gene expression. During this process, a specific segment of DNA is copied into messenger RNA (mRNA) by the enzyme RNA polymerase. This step is crucial as it determines which genes are expressed at any given time.
The key characteristic of transcription is its specificity. Only particular genes necessary for cellular function are transcribed. This selectivity helps regulate cellular activities efficiently, making transcription a beneficial choice in understanding cellular responses to different stimuli. One unique feature of transcription is its ability to produce various RNA forms, such as mRNA, tRNA, and rRNA, from a single DNA template. The advantage here lies in the diversity of RNA molecules produced, each serving distinct functions in the cell.
However, transcription can also result in errors, leading to dysfunctional proteins. This possibility poses a disadvantage, as these errors may contribute to disease processes. Understanding these aspects of transcription is vital for researchers aiming to manipulate gene expression for therapeutic purposes.
Translation
Translation is the second step of gene expression, where the information encoded in mRNA is transformed into a polypeptide chain, ultimately folding into a functional protein. Ribosomes facilitate this process, reading the mRNA sequence and using transfer RNA (tRNA) to assemble the corresponding amino acids.
The key characteristic of translation is its role in protein synthesis, which is vital for numerous cellular functions. This aspect underscores why it is beneficial to discuss translation when exploring RNA functions. A unique feature of translation is the involvement of various factors that ensure accuracy and efficiency during protein synthesis. The advantage of this system lies in its ability to produce specific proteins tailored to current cellular needs, ensuring optimal functionality.
On the downside, translation can also face challenges, such as errors during synthesis or misfolded proteins, which can lead to cellular dysfunction. Investigating these potential pitfalls provides insight into how RNA regulation can impact overall health.
Regulatory Functions
Beyond gene expression, RNA holds vital regulatory functions. Different RNA molecules fine-tune gene activity, influencing how proteins are synthesized and controlling gene expression intervals.
MicroRNAs
MicroRNAs (miRNAs) are small non-coding RNA molecules that modulate gene expression post-transcriptionally. They play a significant role in regulating diverse biological processes, including development, cell proliferation, and apoptosis. Their key characteristic is the ability to inhibit mRNA translation or promote degradation, highlighting their beneficial nature in fine-tuning gene expression.
A unique feature of microRNAs is their high specificity; a single miRNA can target multiple mRNAs, allowing for intricate regulation of gene networks. The advantage here is that miRNAs can quickly adjust gene expression in response to environmental changes. Yet, their dysfunction is linked to various diseases, highlighting the disadvantage of potentially disregulated expression leading to pathological conditions.
Long non-coding RNAs
Long non-coding RNAs (lncRNAs) represent another class of regulatory RNA. Unlike miRNAs, lncRNAs can have diverse roles, including chromatin remodeling, transcriptional interference, and serving as scaffolds for protein complexes. Their key characteristic is their length, which can exceed 200 nucleotides, providing them with structural complexity able to interact with multiple cellular components.
This beneficial aspect makes lncRNAs influential in various cellular processes, including embryonic development and cellular differentiation. A unique feature of lncRNAs is their involvement in regulating gene expression across different dimensions, from chromatin to RNA levels. However, research is ongoing to better understand their specific functions, and their complexity can lead to challenges in studying their roles.
In summary, RNA's functions extend well beyond simple information transfer. From transcription to translation and various regulatory roles, RNA molecules are deeply embedded in the fabric of biological processes affecting health and disease. Understanding these functions is vital for unlocking potential therapeutic avenues and advancing biotechnology.
RNA in Biotechnology
RNA plays a crucial role in biotechnological innovations, bridging molecular biology and practical applications. Its versatility is manifested in various forms, from mRNA to small interfering RNA (siRNA). These RNA types are pivotal in driving advancements that are reshaping medical and agricultural landscapes.
mRNA Vaccines
Recent developments
Recent developments in mRNA vaccine technology have garnered immense attention, especially during the COVID-19 pandemic. Companies like Pfizer-BioNTech and Moderna pioneered the rapid development and deployment of mRNA vaccines, demonstrating significant efficacy in preventing severe illness. The key characteristic of these vaccines is their ability to instruct cells to produce a harmless piece of the virus's spike protein, triggering a robust immune response. mRNA vaccines offer a speedy response in times of need, making them a reference point in vaccine research. Moreover, the unique feature of mRNA vaccines is their potential for rapid updates, allowing modifications against emerging variants without starting from scratch. However, some disadvantages exist, such as storage requirements at ultra-low temperatures.
Implications for public health
The implications of mRNA vaccines for public health are profound. Their deployment has shown notable success in controlling viral spread, which contributes to the broader goal of pandemic response and preparedness. A key characteristic here is the ability to generate a strong and lasting immune response with a relatively simple design process. This makes mRNA a valuable asset in vaccine development. Furthermore, the unique feature of widespread vaccination can build herd immunity, enhancing community protection. The challenges to consider include equitable distribution and public trust, which can affect overall uptake and effectiveness.
Gene Editing Technologies
CRISPR-Cas9
CRISPR-Cas9 has emerged as a transformative tool in gene editing, offering unprecedented specificity and ease of use. It allows for precise modifications of DNA, enhancing our understanding of genetic disorders and facilitating potential curative therapies. Its popularity stems from the straightforward mechanism of targeting particular sequences of DNA, minimizing off-target effects. Additionally, the unique feature of CRISPR-Cas9 is its adaptability, capable of not only knocking out genes but also inserting new ones to correct mutations. Despite its advantages, ethical concerns regarding the permanence of genetic editing remain a critical point of discussion.
Role of RNA guide molecules
The role of RNA guide molecules is integral to the efficacy of CRISPR technology. These RNA sequences direct the Cas9 enzyme to specific genomic locations, ensuring precision in gene editing. This specific targeting has opened doors to a variety of applications, from agricultural enhancements to potential therapies for genetic disorders. The distinctive aspect of RNA guide molecules is their ability to be engineered for diverse purposes, making them attractive in research and therapeutic contexts. However, challenges include the potential for unintended consequences if guide RNAs do not correctly map to the target site in the genome.
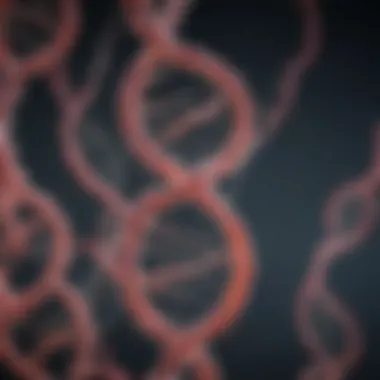
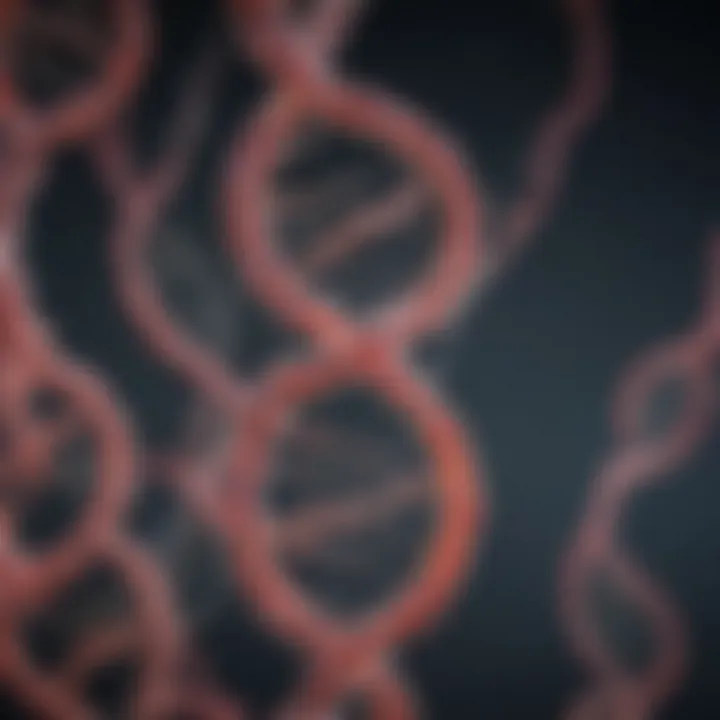
"RNA holds a vital key to unlocking the future of biotechnology, enabling breakthroughs in health and agriculture."
"RNA holds a vital key to unlocking the future of biotechnology, enabling breakthroughs in health and agriculture."
Through these advancements, RNA's importance in biotechnology cannot be overstated. It provides innovative solutions while posing questions that need careful consideration. The continuous exploration of RNA's capabilities promises to reshape the future landscape of biosciences and improve lives globally.
RNA and Disease
The exploration of RNA in the context of disease is crucial for understanding its multifaceted roles in health and pathology. RNA molecules can serve as both markers and mediators of various diseases. Their presence or alteration often indicates disease states, while their functions may directly contribute to disease progression or resilience. In an increasingly RNA-centric world, comprehending these relationships holds great potential for therapeutic advancements.
RNA Viruses
Mechanisms of infection
RNA viruses utilize a range of mechanisms to infect their hosts effectively. A key aspect here is their ability to hijack host cellular machinery, often replicating their RNA genome using the host’s enzymes and resources. This characteristic allows RNA viruses to reproduce swiftly within the host, leading to rapid infection spread.
The unique feature of many RNA viruses is their high mutation rate. This contributes both an advantage and a disadvantage in the study of these viruses. On one hand, the rapid evolution allows the viruses to evade the host's immune responses. On the other hand, this variability also leads to a challenge in developing effective vaccines and treatments. Thus, understanding these mechanisms is vital for creating strategies to combat viral infections, such as those caused by the Influenza virus or Hepatitis C virus.
Examples of RNA viruses
Several RNA viruses have significantly impacted public health. This includes the well-known Human Immunodeficiency Virus (HIV), which causes AIDS. Another example is the Zika virus, which has raised global health concerns due to its link to severe birth defects. The high rate of mutation among these viruses often leads to the emergence of variants that may cause outbreaks.
The significant characteristic of RNA viruses is their ability to rapidly adapt to environmental pressures. This versatility makes them both a focus of concern and a subject of research. The unique features of RNA viruses, primarily their swift transmission and mutation rate, present an intricate challenge that impacts the diagnosis, treatment, and prevention of viral diseases.
RNA in Cancer
Oncogenic RNAs
Oncogenic RNAs have emerged as important figures in the field of cancer research. These RNAs can drive the progression of cancer by promoting uncontrolled cell division and survival. A notable example includes certain microRNAs that, when dysregulated, contribute to tumorigenesis by targeting key tumor suppressor genes.
Highlighting the significant role oncogenic RNAs play within cancer progression makes them a compelling topic for this article. Their unique feature lies in their dual role as both biomarkers and therapeutic targets, offering potential avenues for intervention in cancer treatment.
Potential therapies
Potential therapies targeting RNA have gained traction in recent years. This includes the development of small interfering RNAs (siRNAs) aimed at silencing oncogenic pathways. These therapies offer a precise approach by targeting specific mRNA for degradation, thus halting the synthesis of proteins that promote cancer cell survival.
The key characteristic of RNA-targeted therapies is their specificity. Unlike conventional therapies, which can affect both healthy and cancerous cells, these targeted approaches minimize damage to normal tissues. This unique feature presents advantages in terms of reducing side effects and improving patient outcomes. Nevertheless, logistical challenges such as delivery mechanisms and stability of the RNA therapeutics remain significant hurdles in the field.
In summary, the interrelation of RNA with diseases such as viral infections and cancer underscores its importance in modern biomedical research. By elucidating these complex interactions, significant strides can be made toward enhancing disease diagnosis and developing innovative therapeutic strategies.
Future Directions in RNA Research
The exploration of RNA remains a dynamic area of scientific inquiry. Understanding the future directions in RNA research can illustrate its expanding role in multiple facets of biology. The potential advancements in this field are poised to significantly influence gene therapy, diagnostics, and therapeutic interventions. This section will cover emerging technologies and ethical considerations that arise from ongoing RNA research, providing a glimpse into its prospective impact on future scientific endeavors.
Emerging Technologies
Next-generation sequencing
Next-generation sequencing (NGS) represents a substantial leap in genomic research. This technology enables rapid sequencing of RNA molecules, which helps in understanding gene expression patterns with unprecedented speed and accuracy. One key characteristic of NGS is its ability to produce millions of sequences in parallel. This characteristic makes it a powerful tool in RNA research, as it offers insights into both the coding and non-coding RNA landscape in biological systems.
The unique feature of NGS is its high-throughput capacity. While traditional sequencing methods are limited in the amount of data they can process, NGS can handle vast amounts of RNA samples, making it cost-effective and efficient for large-scale studies.
However, there are some disadvantages to NGS, particularly regarding the complexity of data analysis. The interpretation of NGS results can be challenging and may require advanced computational resources, which can be a limiting factor for some research environments.
RNA interference
RNA interference (RNAi) is another promising area in RNA research. It is a biological process where RNA molecules inhibit gene expression or translation. This mechanism allows researchers to target specific genes, making it a useful tool for gene silencing. The ability of RNAi to selectively knock down genes has made it increasingly popular in functional genomics and therapeutic applications.
One key characteristic of RNA interference is its precision. By utilizing small interfering RNA (siRNA) or microRNA (miRNA), researchers can manage gene expression with accuracy. This precision makes RNAi an essential method in both basic research and potential clinical applications.
The unique feature of RNA interference lies in its versatility. It can be applied to various organisms and cell types, making it a flexible research tool. However, there are challenges, including potential off-target effects, where unintended genes may also be silenced, leading to undesired outcomes.
Ethical Considerations
Gene editing ethics
The ethical implications surrounding gene editing technologies, such as CRISPR-Cas9, are paramount as RNA research progresses. The ability to modify genetic sequences raises questions about consent, potential misuse, and long-term impacts on future generations. One important characteristic of gene editing ethics is the necessity of balancing scientific innovation with moral responsibility. Addressing this balance is crucial for maintaining public trust in scientific advancements.
The unique aspect of gene editing ethics is its interdisciplinary nature, encompassing scientific, legal, and social considerations. It requires ongoing dialogue among scientists, ethicists, policymakers, and society. However, navigating these discussions can be complex and may slow the adoption of beneficial technologies.
RNA-based therapies
RNA-based therapies are becoming increasingly significant as therapeutic options evolve. Therapies targeting RNA aim to rectify disease-causing genetic defects. One key characteristic here is their potential for personalized medicine, as RNA therapies can be tailored to specific genetic profiles. This capability positions them as powerful tools in combating a range of diseases, including genetic disorders and cancers.
The unique feature of RNA-based therapies is their adaptability. These therapies can be designed to target various pathways and mechanisms in cells. However, alongside their promise come challenges, including delivery mechanisms and immune responses. These factors must be addressed to maximize their therapeutic effects while minimizing adverse reactions.
Further exploration of the RNA field holds untold potential. The enhancement of both emerging technologies and ethical considerations will be pivotal for the responsible advancement of RNA research.
Further exploration of the RNA field holds untold potential. The enhancement of both emerging technologies and ethical considerations will be pivotal for the responsible advancement of RNA research.