Exploring the Complex Cells of the Nervous System
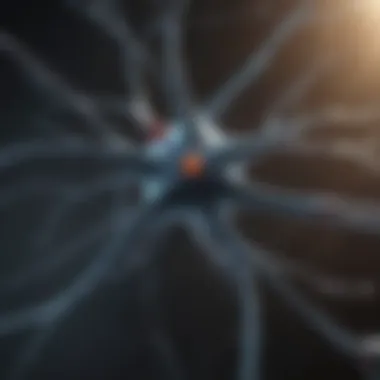
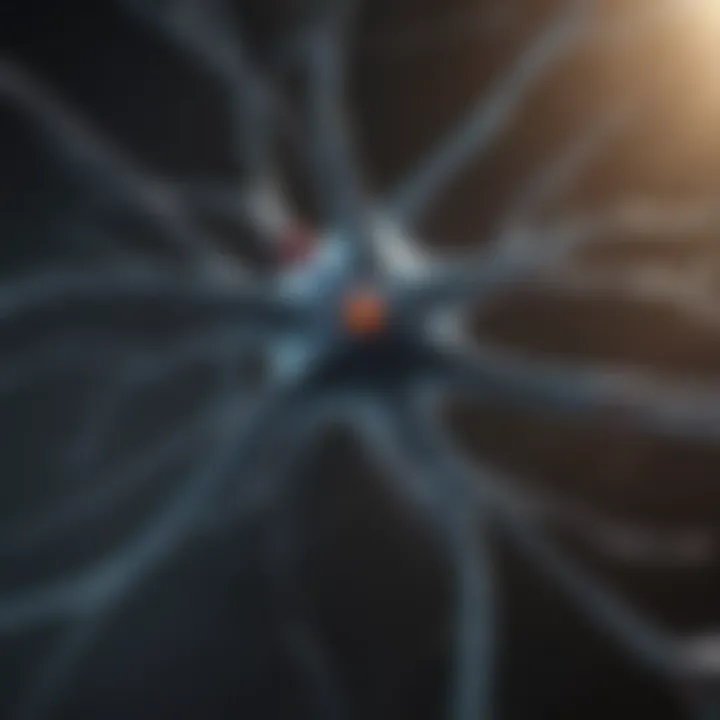
Intro
The nervous system is a complex and sophisticated network that serves as the body's command center. Its architecture is built upon various specialized cells, each playing pivotal roles in maintaining the overall functionality of this critical biological system. Understanding these cells is fundamental for students, educators, researchers, and professionals alike, as it sheds light on both normal physiological processes and various neurological disorders that can arise from their dysfunction.
While many might picture neurons as the stars of the show, glial cells are equally essential, providing support, nourishment, and protection. Neurons communicate through electrical impulses, but without the quiet yet crucial assistance of glial cells, the whole system would likely stumble and fall apart. This article intends to dive deep into the intricate balance of these cells, their significance in health, and the consequences of their failure.
Key Concepts
Definition of the Main Idea
The nervous system can essentially be seen as a vast communication network that relies on two primary cell types: neurons and glial cells.
- Neurons: Responsible for transmitting signals, neurons are equipped with distinctive structures such as dendrites and axons that facilitate communication across synapses. They can be thought of as wires in an electrical circuit, sending and receiving messages that dictate everything from muscle movement to sensory perception.
- Glial Cells: Traditionally viewed as the supportive framework of the nervous system, glial cells are anything but passive. They actively participate in processes like maintaining homeostasis, providing insulation for neurons, and even contributing to the repair of nervous tissue after injury.
This dual-cell focus paves the way to a layered understanding of neurological health and disease.
Overview of Scientific Principles
The interaction between neurons and glial cells highlights a theme in neuroscience: collaboration and communication. Here are some scientific principles to consider:
- Action Potentials: Neurons communicate through action potentials—rapid changes in electrical charge that travel along axons. This is akin to a game of telephone, where precision is key for the correct message to reach its destination.
- Synaptic Transmission: At synapses, neurotransmitters are released from one neuron and bind to receptors on another, facilitating communication that’s critical for learning, memory, and other cognitive functions.
- Homeostasis and Support: Glial cells maintain the environment around neurons, regulate nutrient exchange, and respond to injury by managing inflammation and the cleanup of cellular debris. They ensure that neurons can operate smoothly and effectively.
The structures and functions of these cells provide foundational knowledge that informs everything from basic biology to complex medical discussions. Understanding them is like having the blueprint to a building; it reveals how each part works together to create a solid structure, with potential vulnerabilities noted throughout.
"The nervous system is like a symphony orchestra, where each musician, whether a neuron or a glial cell, plays a unique role in creating a beautiful melody of cognition and functionality."
"The nervous system is like a symphony orchestra, where each musician, whether a neuron or a glial cell, plays a unique role in creating a beautiful melody of cognition and functionality."
Current Research Trends
Recent Studies and Findings
As neuroscience continues to evolve, researchers are uncovering intricate relationships within the nervous system:
- Recent studies have demonstrated that glial cells are more than just supportive elements; they show intelligence and responsiveness akin to neurons, indicating a greater role in brain communication.
- Investigations into neuroinflammation have revealed that certain types of glial cells can contribute to neurological diseases like Alzheimer's and multiple sclerosis.
Significant Breakthroughs in the Field
Breakthroughs in neurobiology and genomics are reshaping our understanding of nervous system cells:
- Findings have indicated that manipulating specific signaling pathways in glial cells could offer new therapeutic avenues for treating neurodegenerative conditions.
- Advancements in imaging technologies allow for real-time observation of neuronal-glial interactions, leading to insights into how these cells cooperate during both normal and pathophysiological conditions.
By diving into these trends, readers can grasp larger implications for both fundamental and clinical neuroscience. This knowledge could guide future exploration and therapeutic innovation. Understanding the cellular landscape of the nervous system allows for informed expectations about health, disease, and the future of neurobiology.
Preface to the Nervous System Cells
The nervous system operates as a complex network, orchestrating everything from basic reflexes to higher cognitive functions. Understanding the cells that make up this system is crucial. Each cell plays a distinct role, whether in transmitting signals or providing support and nourishment. These cells, primarily neurons and glial cells, form the backbone of the nervous system, and their intricate relationships dictate the overall health and functionality of our minds and bodies.
Overview of the Nervous System
The nervous system can be split into two main parts: the central nervous system (CNS), which consists of the brain and spinal cord, and the peripheral nervous system (PNS), which connects the CNS to the rest of the body. Within this framework, cells communicate through electrical and chemical signals, forming a sophisticated relay system. For instance, sensory information from the body travels to the brain via sensory neurons, while motor neurons send signals back out to execute a response.
- The CNS is where processing occurs, integrating information and coordinating responses.
- The PNS ensures that communication to and from the CNS is efficient, impacting reflex actions, muscle control, and sensory perception.
These interconnections highlight why an understanding of these cellular components is vital; any disruption can lead to a myriad of health issues, including neurological disorders.
Importance of Cell Types in Nervous System
Every cell in the nervous system has its unique function and significance. Neurons are often regarded as the stars of the show, facilitating communication through synapses, where neurotransmitters are released. They can be sensory, motor, or interneurons, making them versatile components in this intricate dance of signals.
On the other hand, glial cells—often overlooked—provide essential support. They help maintain homeostasis, clean up debris, and even assist in the regeneration of neurons after injury.
- Neurons
- Glial Cells
- transmit signals
- manage communication
- support and protect neurons
- play key roles in repair and maintenance
Together, they ensure that the nervous system operates smoothly. Thus, understanding not just what each type of cell does but also how they collaborate is fundamental. Disruption in any of these roles can lead to disorders ranging from Alzheimer’s disease to Multiple Sclerosis, underlining the importance of continued research into these cellular components.
"The intricacy of cellular interactions in the nervous system is a testament to the complexities of life itself."
"The intricacy of cellular interactions in the nervous system is a testament to the complexities of life itself."
As we dive deeper into the topic, we’ll explore the specific types of neurons and glial cells, their structures and functions, and how they interact to maintain the health of the nervous system.
Neurons: The Fundamental Units
Neurons are the powerhouses of the nervous system, serving as the primary units responsible for transmitting information throughout the body. Their role is paramount, as they facilitate communication between different parts of the system, making them essential for both simple reflexes and complex cognitive processes. Understanding neurons, their structure, and function is crucial in appreciating how intricately our bodies react to stimuli and maintain homeostasis. In this section, we will explore the various components of neurons, the distinct types of neurons, and how they communicate.
Structure of Neurons
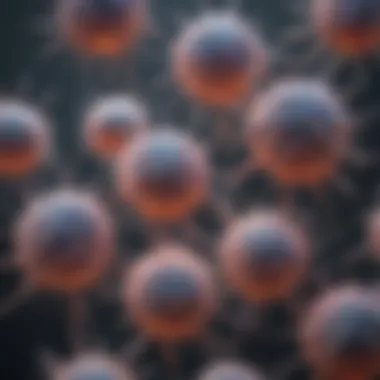
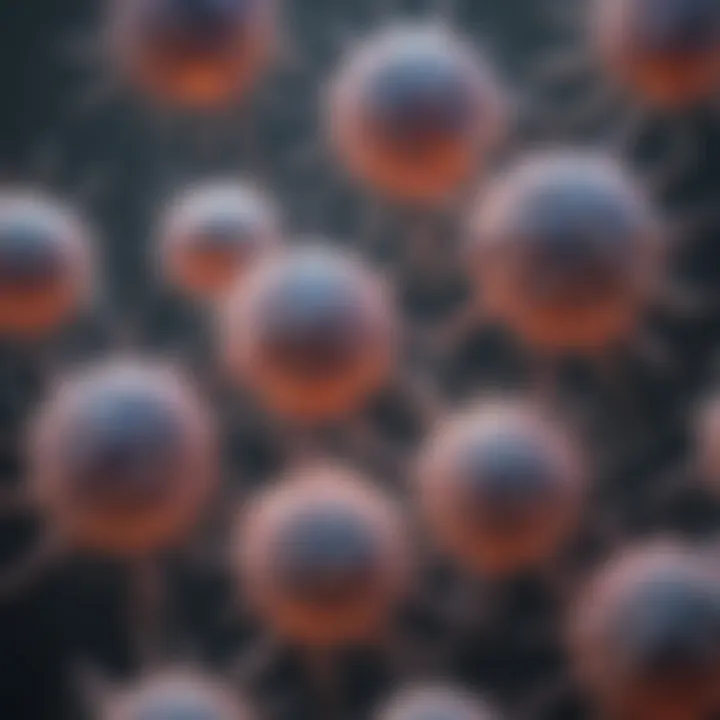
The structure of neurons is tailored for their function. Comprising three main parts: soma, axon, and dendrites, each component plays its indispensable role in the operational efficiency of neurons.
Soma
The soma, often referred to as the cell body, contains the nucleus and is where essential cellular processes happen. One key characteristic of the soma is its rich content of mitochondria and ribosomes, which are vital for energy production and protein synthesis. This feature makes the soma a popular aspect of neuron study, as it underlines how neurons sustain their high metabolic demands.
A unique attribute of the soma is its role in integrating signals received from dendrites. This integration is crucial because it determines whether or not a neuron will fire an action potential. However, while vital, the soma isn't involved in signal transmission; thus, its function can seem somewhat secondary compared to the axon and dendrites.
Axon
The axon is the long, slender projection of the neuron that conducts electrical impulses away from the soma. It’s notable for its ability to transmit signals over considerable distances, sometimes even up to a meter in length. This characteristic makes the axon a fundamental feature of neuron functionality, as it connects different parts of the nervous system.
What sets the axon apart is the myelin sheath, a fatty layer that insulates it and enhances the speed of signal transmission. This insulation is instrumental in facilitating rapid communication within the nervous system. One disadvantage, however, lies in the vulnerability of unmyelinated regions; action potentials can slow down, making the nervous system less efficient.
Dendrites
Dendrites are the branching structures that extend from the soma and receive incoming signals from other neurons. Their tree-like arrangement increases the surface area available for synaptic connections. A significant characteristic of dendrites is their plasticity—their ability to change in structure, which is associated with learning and memory.
Dendrites are beneficial in expanding the reach of a neuron, allowing it to form connections with multiple different neurons. However, they are also the sites where signal attenuation can occur, meaning that some of the incoming signals might weaken before reaching the soma, possibly affecting the neuron's overall responsiveness.
Types of Neurons
Neurons can be categorized based on their function into three main types: sensory neurons, motor neurons, and interneurons. Each type plays a unique and vital role in processing information and facilitating responses.
Sensory Neurons
Sensory neurons are responsible for taking information from the surrounding environment and converting it into electrical impulses that can be interpreted by the brain. Their primary characteristic is their specialized endings—these can react to stimuli such as light, sound, and touch, thereby playing a crucial role in perception.
One benefit of sensory neurons is that they provide the necessary information for decision-making and reaction. However, a unique feature of sensory neurons is their susceptibility to damage, which can lead to sensory deficits, reflecting how critical they are in conveying real-time information from our environment.
Motor Neurons
Motor neurons transmit signals from the central nervous system to muscles and glands, facilitating movement and physiological responses. Their primary characteristic includes long axons that can connect to multiple muscle fibers, allowing for coordinated movements.
A notable advantage of motor neurons is their ability to efficiently translate electrical impulses into mechanical actions. However, this function can also be a drawback, as damage to motor neurons can result in paralysis or loss of muscle control, illustrating how essential they are for bodily functions.
Interneurons
Interneurons serve as connectors within the nervous system, facilitating communication between sensory and motor neurons and processing information. Their distinguishing feature is their vast array and intricate networks, often found in the spinal cord and brain.
The primary benefit of interneurons lies in their ability to perform complex integrations and reflex actions. However, due to their diverse roles, they can also be involved in disorders like anxiety and mood disorders when malfunctioning, emphasizing their significance in maintaining homeostasis.
Neuronal Communication
The transmission of information between neurons is predominantly defined by synaptic transmission, coupled with the chemical substances known as neurotransmitters.
Synaptic Transmission
Synaptic transmission refers to the process wherein neurons communicate with each other through synapses, which are the points of contact where neurotransmitters are released. A key characteristic of this process is that it allows for rapid and targeted communication between neurons.
One advantage of synaptic transmission is that it enables the nervous system to process information efficiently, but it can also be influenced by various factors such as drugs, potentially leading to changes in behavior or cognition.
Neurotransmitters
Neurotransmitters are chemicals that transmit signals across a synapse from one neuron to another. Their main characteristic is their specificity; different neurotransmitters affect different types of neurons and even regions of the brain.
A unique feature of neurotransmitters is their role in various bodily functions, from mood regulation to muscle contractions. However, an imbalance in neurotransmitter levels can result in various psychological disorders, highlighting their crucial role in maintaining neurological health.
Glial Cells: Beyond Support
Glial cells, sometimes viewed as mere support staff in the grand narrative of the nervous system, actually play multifaceted roles that extend far beyond simply holding everything together. Despite not conducting signals like neurons, glial cells are critical in maintaining a healthy environment for neurons, promoting communication, and facilitating repair processes following injury. They are enablers, often working behind the curtain yet always on duty, ensuring that the nervous system functions optimally. Their importance cannot be overstated, and exploring the various types of glial cells leads us to understand their complex yet crucial contributions in more depth.
Types of Glial Cells
Astrocytes
Astrocytes are star-shaped glial cells and play several roles. Their main job is to maintain the balance of ions and nutrients in the extracellular space; think of them as the custodians of neural surroundings. One key characteristic of astrocytes is their ability to form a blood-brain barrier. This barrier is significant, as it protects the brain from potentially harmful substances in the bloodstream. Because of their unique structure and functions, astrocytes are a popular point of interest in this article. They help regulate blood flow and ensure neurons receive adequate oxygen and glucose. The challenge, however, lies in their ability to respond to injury, sometimes leading to a problematic scarring process, which might have negative impacts on neuronal function.
Oligodendrocytes
Next up are oligodendrocytes, which are essential for myelination in the central nervous system. These cells produce myelin, a fat-like substance that wraps around neuronal axons, acting like insulation for electrical conductance. The efficiency of signal transduction greatly improves due to this myelin sheath. A prominent feature of oligodendrocytes is that a single cell can extend its processes to multiple axons, a fact that speaks volumes of their efficiency. This makes oligodendrocytes a critical focus in discussions surrounding nerve injuries and diseases such as multiple sclerosis. However, when oligodendrocytes fail, it can lead to communication failures in neuronal circuits, which can profoundly affect behavior and cognition.
Microglia
Microglia are often described as the brain's immune responders. These cells constantly monitor the environment, ready to respond to disease or injury, and they serve to clear out debris and dead neurons. A key feature of microglia is their ability to transform from a surveillance mode to an activated state when inflammation occurs, which is crucial for disease response. Their role as janitors of the brain cannot be overlooked; however, the downside is that their activation can sometimes result in chronic inflammation, which may further exacerbate neurological disorders. Hence, microglia embody both essential support and potential complication in the realm of nervous system health.
Functions of Glial Cells
Structural Support
The structural support provided by glial cells is fundamental for maintaining the overall integrity of the nervous system. They create frameworks that not only hold neurons in place but also guide their growth. This characteristic makes glial cells indispensable for neurogenesis and injury repair. The unique advantage here is that providing such structural scaffolding enables complex network formations necessary for effective communication between neurons. Yet, if this structural support becomes excessive, it may cause problems like scarring tissue that blocks neuronal repair.
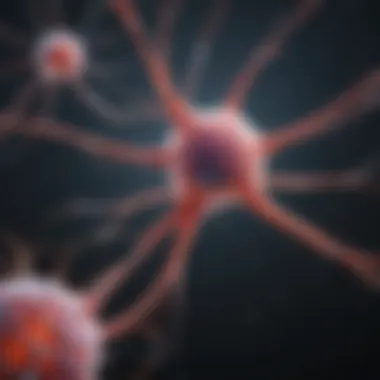
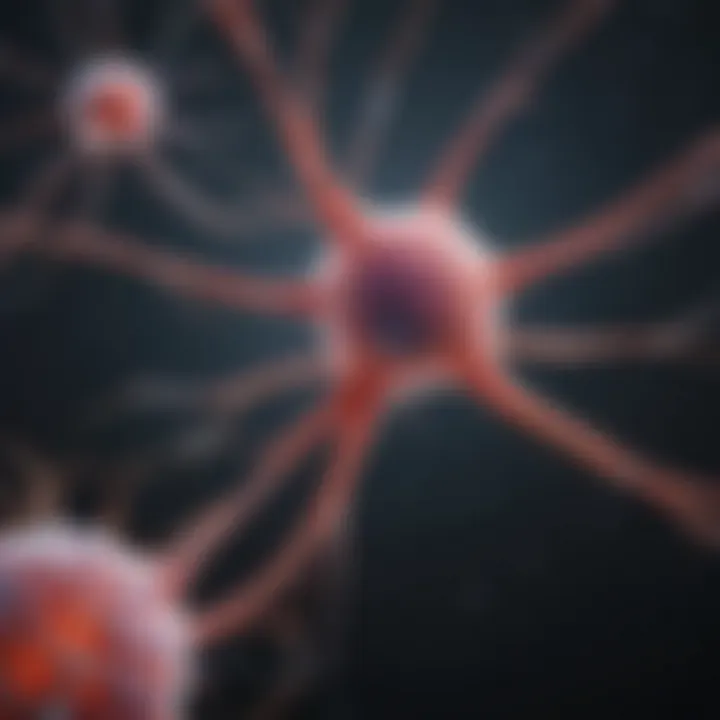
Nutrient Supply
Glial cells also ensure that neurons get their 'three square meals a day' – and much more! Their role in nutrient supply is vital, as they facilitate transport of glucose, potassium ions, and neurotransmitter precursors from blood to neurons. This aspect is beneficial as it optimizes neuronal functioning and energy levels. However, nutrient supply must be closely regulated; any imbalance might lead to neuronal dysfunction or even cell death, which can have dire consequences.
Waste Clearance
A lesser-known but nearly equally important function of glial cells is waste clearance. They help clear metabolic byproducts such as excess neurotransmitters or damaged cellular components from the extracellular environment. This purification is crucial for maintaining homeostasis within the neural circuit. The advantage of waste clearance is clear: it prevents toxic accumulation and potential neurodegeneration. Conversely, if waste clearance mechanisms are compromised, toxic substances can build up, resulting in neuronal damage over time.
Interactions Between Neurons and Glial Cells
Communication Mechanisms
The communication between neurons and glial cells is a symbiotic relationship where both parties benefit. Astrocytes and oligodendrocytes communicate with neurons through signaling molecules that can influence neuronal activity. This interaction is beneficial because it fine-tunes neurotransmission and synaptic efficacy. Yet, it does present a challenge; if signaling is disrupted, it can lead to a breakdown in communication, potentially resulting in neurological disorders.
Role in Neurodevelopment
During neurodevelopment, glial cells guide neurons to their proper locations, thus profoundly impacting neural network formation. Their role in neurodevelopment is crucial for ensuring that the brain develops appropriately. The unique feature here is their involvement in guiding neuronal migration and differentiation. However, if glial cells misbehave, it can lead to developmental disorders, emphasizing the necessity of understanding these dynamics in both typical and atypical development.
Glial cells play pivotal roles in ensuring the health and functionality of the nervous system. Understanding their complex interactions will pave the way for innovative therapeutic strategies in treating neurological disorders.
Glial cells play pivotal roles in ensuring the health and functionality of the nervous system. Understanding their complex interactions will pave the way for innovative therapeutic strategies in treating neurological disorders.
The Role of Myelin in Nervous System Function
Myelin is a crucial player in the efficient functioning of the nervous system. This fatty substance wraps around the axons of neurons like a protective sheath, enhancing the speed and efficiency of electrical impulses. Without myelin, communication between nerve cells would be compromised, leading to significant consequences for both sensory and motor functions.
What is Myelin?
Myelin is primarily composed of lipids and proteins. This unique composition allows it to create a fatty layer that insulates nerve fibers. This insulation is vital; it prevents electrical signals from dissipating and ensures that they travel rapidly along the axon. Each segment of myelin is separated by small gaps known as nodes of Ranvier. These gaps facilitate a process called saltatory conduction, where the signal jumps from one node to the next, greatly increasing transmission speed. Essentially, myelin can be thought of as the electrical tape that keeps things running smoothly in the nerve environment.
Myelination Process
Myelination occurs during the development of the nervous system, starting during childhood and continuing into young adulthood. Specialized cells are responsible for creating myelin: oligodendrocytes in the central nervous system and Schwann cells in the peripheral nervous system. The process begins with these glial cells extending their membranous projections to wrap around the axon multiple times. This layered buildup results in the formation of a robust myelin sheath that provides necessary support. It’s like building a fortress around a vital pathway to ensure secure and rapid communication.
Impact of Demyelination
Demyelination refers to the loss or damage of myelin. This can lead to a host of neurological problems. When the myelin sheath is compromised, the speed of nerve impulses is slowed or completely blocked, which can severely impact bodily functions.
Multiple Sclerosis
Multiple sclerosis (MS) is one of the most well-known consequences of demyelination. It occurs when the immune system mistakenly attacks the myelin in the central nervous system. This can lead to symptoms such as muscle weakness, coordination problems, and neurological deterioration. The key characteristic of MS is its unpredictable pattern of flare-ups and remissions, making it a complex condition to manage. MS provides a prime example of the importance of myelin; as the protective layer is damaged, communication within the nervous system is significantly disrupted, leading to varied and often debilitating symptoms. Understanding MS can enhance our insight into how critical myelin is for overall nervous function.
Other Conditions
Demyelination is not solely tied to multiple sclerosis. Other conditions, such as Guillain-Barré syndrome, also highlight the consequences of myelin loss. In Guillain-Barré, the body’s immune system attacks the peripheral nerves, leading to muscle weakness and sometimes paralysis. The unique feature of Guillain-Barré is that it can occur suddenly, often following an infection. This characteristic sets it apart from other chronic demyelinating illnesses and showcases the intricate interplay between the immune system and neurological health. Exploring various conditions associated with demyelination can further emphasize the critical role that myelin plays in maintaining proper neurological function.
Understanding myelin and its implications not only aids in appreciating the complexity of the nervous system but is also essential in guiding future therapeutic interventions.
Understanding myelin and its implications not only aids in appreciating the complexity of the nervous system but is also essential in guiding future therapeutic interventions.
Overall, myelin's role cannot be overstated; it is foundational for efficient nervous system function and significantly impacts our understanding of neurological disorders.
Cellular Response to Injury
The cellular response to injury constitutes a pivotal field of study within neuroscience. Understanding how the cells of the nervous system react to damage reveals much about recovery processes, healing capabilities, and the potential for regeneration. This topic sheds light on the resilience of neurons and glial cells, emphasizing the necessity of these responses for overall nervous system health and functionality.
After such injury, whether from trauma, disease, or other insults, both neurons and glial cells engage in complex responses aimed at restoring stability. This process can highlight significant adaptive mechanisms, showing how the body can strive to maintain its integrity amid disruption. It also opens up discussions about regenerative medicine, where enhancing these cellular responses could lead to breakthroughs in treating neurological disorders.
Neuronal Regeneration
Neurons possess a complicated relationship with regeneration. Unlike other cell types in the body, mature neurons typically have limited intrinsic capacity for regeneration after injury. However, certain peripheral neurons display a surprising ability to regenerate their axons if the injury is not too extensive.
Research highlights that factors contributing to successful neuronal regeneration include:
- The type of neuronal injury.
- Environmental conditions surrounding the injury site.
- The influence of glial cells in the regeneration process.
This balance of regeneration and scarring is crucial. If the conditions aren't conducive, neuronal loss can lead to permanent deficits and neurological dysfunction. Finding ways to harness and enhance neuronal regeneration remains a key focus in contemporary neuroscience research.
Glial Cell Activation
Glial cells, vastly outnumbering neurons in the central nervous system, play a key role in reparative processes following an injury. Their activation can lead to several responses aimed at supporting neuronal survival and healing.
Reactive Astrogliosis
Reactive astrogliosis is a hallmark of glial response to injury and is characterized by the transformation of astrocytes into a reactive state. This reaction has both beneficial and adverse aspects. On one hand, reactive astrocytes help maintain homeostasis, provide support for neurons, and promote the repair of the extracellular matrix.
Conversely, an excessive astrocytic response can lead to glial scarring, which brings challenges to neuronal regeneration.
A key characteristic that makes reactive astrogliosis vital in this discussion is its actve role in modulating inflammation. Astrocytes take on a protective function by releasing various neurotrophic factors that can be incredibly supportive during recovery. However, the inability to manage their reactivity can also contribute to persistent issues in nervous system health, which need to be carefully balanced.
Microglial Response
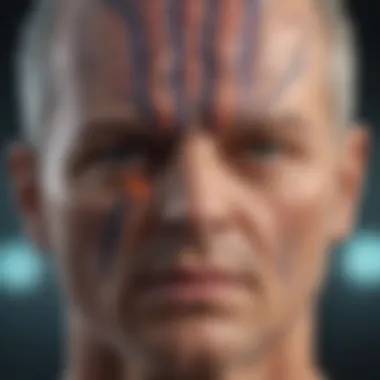
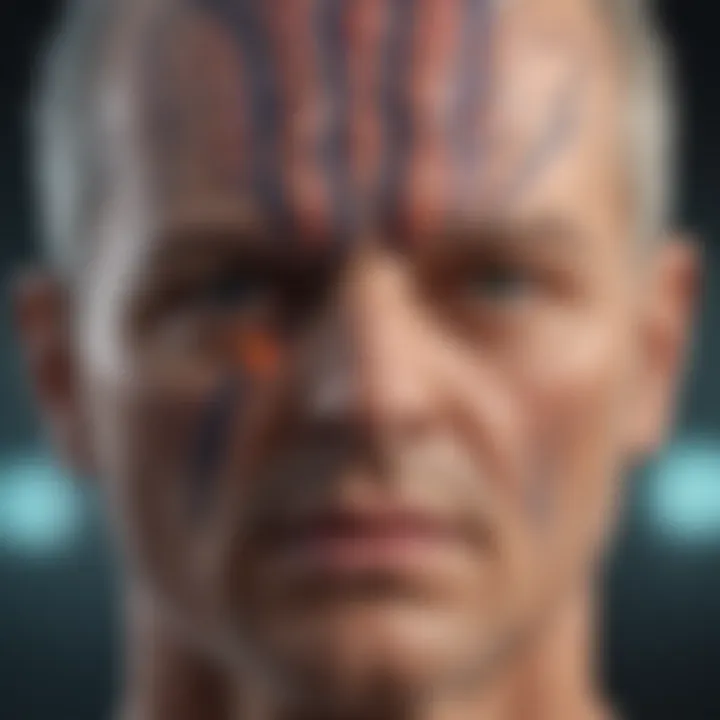
Microglia act as the brain's resident immune cells, quickly responding to neuronal injury or infection. They undergo a dramatic transformation when activated, evolving from a resting state into an active form that can engulf debris and signal to neighboring cells. This aspect is crucial for clearing dead cells and preventing secondary damage through inflammation.
The notable characteristic of microglial response is their role in maintaining neuronal integrity. While they are essential for clearing out harmful materials, their activation must be regulated. Over-activation can facilitate chronic inflammation, potentially damaging healthy neurons.
In summary, both reactive astrogliosis and the microglial response are integral to understanding the broader context of the nervous system’s healing capabilities. These mechanisms reveal how the system strives to heal itself while also showing the fine line between beneficial support and detrimental overreaction.
Neurological Disorders Related to Cellular Dysfunction
Understanding neurological disorders is crucial to grasp the full picture of how cellular dysfunction impacts the nervous system. These disorders arise mainly when the delicate balance of neuronal and glial functions is disrupted. Focusing on this topic allows us to unveil the complex interactions between different cell types and how their malfunction can lead to devastating consequences for individuals. The significance of this exploration provides insights not just for the affected individuals but also for broader medical and research communities striving to develop effective treatments.
Common Neurological Disorders
Alzheimer's Disease
Alzheimer's Disease is perhaps one of the most discussed neurological disorders today. A distinguishing facet of this disease is the gradual loss of memory and cognitive function. It primarily manifests in the elderly, but the underlying biological disruptions are far from straightforward. This disorder serves as a valuable example in this article due to its multifaceted nature, which encapsulates the interplay of neuron degradation and glial cell dysfunction.
One key characteristic of Alzheimer's is the presence of amyloid-beta plaques and tau tangles, which severely impair neuronal communication. The unique feature of the disease is its chronicity; it unfolds over many years, allowing for significant contributions to research focused on neurodegeneration and cellular repair strategies.
The advantages of exploring Alzheimer's in this article stem from its relevance to a wide audience. By delving into the implications of amyloid plaques or tau tangles, we not only elucidate the pathology but also highlight potential avenues for therapeutic intervention. This focus on Alzheimer's makes it a pertinent choice, allowing readers to appreciate the urgency behind understanding cellular dysfunction.
Parkinson's Disease
Parkinson's Disease, another prevalent disorder, presents uniquely in how it affects motor control. It predominantly involves the degeneration of dopaminergic neurons in the substantia nigra, leading to symptoms such as tremors and rigidity. This aspect is vital, as it encapsulates the essence of how neuronal loss translates to functional deficits.
What makes Parkinson's notable in this discussion is its characteristic motor symptoms, which contrast sharply with the cognitive decline seen in Alzheimer's. This divergence in symptoms allows for a richer discussion about cell-type specificity in relation to dysfunction. A unique feature of Parkinson's is its gradual progression and the heterogeneous manifestation of symptoms among patients, making research complicated yet fascinating.
Analyzing Parkinson’s contributes valuable insights into the intricate dynamics of glial cell interactions and neuronal survival, offering a look at how restoring dopaminergic function might mitigate symptoms. Including Parkinson’s in this article provides a comprehensive overview of how different disorders reflect the broader theme of cellular dysfunction and its implications on health.
Impact of Glial Cell Dysfunction
The role of glial cells in neurological disorders is crucial yet often overshadowed by the focus on neurons. Glial cell dysfunction can significantly alter the homeostasis of the brain environment, impacting not just neuronal health but also the entire nervous system function. For instance, in Alzheimer's, activated astrocytes can have both protective and harmful roles, leading to inflammation and exacerbation of neuronal damage. Micorglia have a double-edged sword effect, where their activity may either clear debris or contribute to neuroinflammation, depending on the context.
Understanding glial cell dysfunction offers promising avenues for therapeutic interventions. By restoring their normal functions, we might find ways to counteract or even potentially reverse the effects of various disorders.
"Neural health is not just about neurons; glial cells are equally important in maintaining balance."
"Neural health is not just about neurons; glial cells are equally important in maintaining balance."
Future Directions in Nervous System Research
Exploring the future directions in nervous system research is crucial for understanding how we can enhance and protect the functionality of the brain and its components. As we continue to learn more about the complexities of neural cells, there's a pressing need to focus on innovative approaches that bridge the gaps in our knowledge. With advances in technology and therapeutic strategies, researchers are venturing into new territories that were once considered mere science fiction.
Emerging Therapies
Emerging therapies in neural research reflect the dynamic nature of medical science. These therapies are often at the intersection of biology and technology, aiming to restore, replace, or regenerate damaged neurons and glial cells. For instance, stem cell therapies have gained attention for their potential to regenerate nerve tissue in conditions like spinal cord injuries and degenerative diseases. Furthermore, gene therapy is being explored as a means to correct genetic defects that lead to neurological disorders.
What's particularly fascinating is that these therapies are not only about fixing damage but also about enhancing natural functions. Recent studies suggest that precision medicine, which tailors treatment based on individual genetics and environment, might revolutionize how we address disorders. This personalized approach signifies a shift from conventional one-size-fits-all strategies toward more customized solutions, improving outcomes for patients.
Technological Advances
Advancements in technology play a significant role in the evolution of nervous system research. They provide tools and methodologies that enhance our understanding and treatment of neural conditions.
Neuroimaging
Neuroimaging techniques, such as functional MRI (fMRI) and positron emission tomography (PET), allow scientists to visualize brain activity in real-time. This non-invasive observational power is a key characteristic of neuroimaging, helping researchers identify which areas of the brain are involved in specific functions and how various disorders may affect those areas. The clear visualization it provides makes neuroimaging a popular choice in both research and clinical settings.
Unique features of neuroimaging include:
- Real-time tracking of brain activity: This offers insights into how the brain functions during various tasks.
- Safety: It is non-invasive, minimizing risk to patients.
However, neuroimaging does have its limitations. For instance, the resolution of fMRI can be inadequate for observing finer neural pathways or structures. Moreover, interpreting the results can be complex, often requiring sophisticated statistical analysis.
Genetic Studies
Genetic studies have become a focal point in understanding the basis of neurological disorders. The integration of genetics into neuroscience, known as neurogenetics, is pivotal in unraveling the hereditary aspects of diseases like Alzheimer's and Autism Spectrum Disorder. One of the defining features of genetic studies is their capacity to link specific genetic variations to increased risk for certain conditions.
This offers several advantages, such as enabling early identification of at-risk individuals, which can lead to preventive strategies before the onset of symptoms. Additionally, genetic studies can help uncover new targets for drug development, providing a pathway towards innovative treatment options. Yet, there are challenges here as well. The complexity of gene-environment interactions can complicate findings, and ethical concerns around genetic data usage persist.
Epilogue
The conclusion serves as a pivotal part of this article, tying together the threads of knowledge presented throughout the various sections and underscoring the significance of comprehending the cells of the nervous system. Grasping the complexities of neurons and glial cells not only enhances our understanding of their unique roles but also sheds light on the intricate web of interactions that sustain overall brain health and function.
Summary of Key Points
- Diverse Cell Types: Neurons, as the fundamental units of the nervous system, and glial cells, serving as their support and protectors, are both integral to its functionality. Each type has specialized roles—neurons transmit information while glial cells provide structural, nutritional, and protective support.
- Impact of Dysfunction: The article emphasizes that malfunctions within these cells can lead to severe neurological disorders. Conditions like Alzheimer's disease and Parkinson's disease illustrate how dysfunction not only affects individuals but also presents a broader challenge to public health.
- Emerging Research and Technologies: The exploration of new therapies and technological advancements like genetic studies and neuroimaging paves the way for future insights, potentially transforming approaches to treatment.
- Importance of Communication: Understanding the communication mechanisms among neurons and glial cells highlights the dynamic nature of the nervous system, demonstrating that the health of one can influence the other.
Final Thoughts on Nervous System Cells
In wrapping up our exploration, it's essential to appreciate that the nervous system is an ever-evolving field of study. The interactions between cell types are not just functional but also indicative of a larger narrative about health and disease. As we delve deeper into the realm of neurology, the implications of our findings can lead to significant advancements in treating disorders and providing insight into the fundamental aspects of human thought and behavior.
The journey of understanding these cellular components, hence, isn't merely academic; it's a bridge to comprehending our very identities and the nature of our interactions with the world.
"In science, we often take for granted the small building blocks of life. However, it is through the lens of the cellular world that we uncover the threads that weave the fabric of existence."
"In science, we often take for granted the small building blocks of life. However, it is through the lens of the cellular world that we uncover the threads that weave the fabric of existence."
This knowledge not only impacts academic and clinical settings but also resonates with every individual, illustrating how the microscopic can influence the macroscopic in profound ways.