Exploring Plasmonic Nanoparticles: Fundamentals & Uses
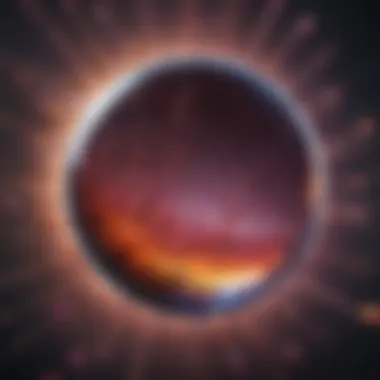
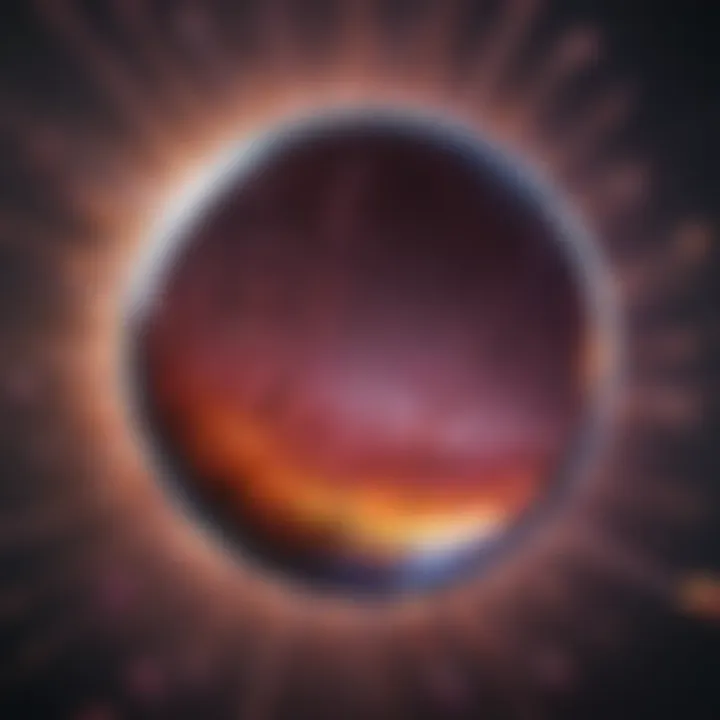
Intro
Plasmonic nanoparticles have gained significant attention within the scientific community due to their distinctive optical characteristics. These nanoparticles exhibit phenomena linked to the collective oscillation of conduction electrons. Understanding the intricate principles governing these behaviors is essential for exploiting their potential in various applications, from enhancing spectroscopic techniques to advancing therapeutic methods.
This article aims to meticulously explore these nanoparticles, starting with foundational concepts, progressing to current research trends, and ultimately unveiling their broad applications. Scientists and researchers working in fields such as nanotechnology, optics, and biology will find this discourse particularly useful.
Key Concepts
Definition of the Main Idea
Plasmonic nanoparticles are nanoscale particles made from noble metals, predominantly gold and silver. These materials can support localized surface plasmon resonances, which occur when light interacts with the conduction electrons at the surface of the nanoparticles. This interaction leads to enhanced electromagnetic fields around the particles, producing striking optical effects, such as strong light scattering and absorption.
Overview of Scientific Principles
The underlying science of plasmonics is rooted in electromagnetic theory. When light encounters a metal nanoparticle, some of the light's energy excites the conduction electrons, causing them to oscillate collectively. The collective oscillation is known as the plasmon, which can resonate at specific frequencies depending on factors such as size, shape, and material of the nanoparticles.
This resonance results in several optical phenomena, including:
- Enhanced absorption and scattering of light
- Colorimetric changes
- Field enhancement in surface-enhanced Raman scattering (SERS)
The properties of these nanoparticles are influenced by their geometrical and chemical characteristics. In practical terms, this means that careful design of the nanoparticles can tailor their behavior for specific applications.
Current Research Trends
Recent Studies and Findings
Recent advances in the synthesis and functionalization of plasmonic nanoparticles have paved the way for groundbreaking research. Methods like chemical reduction, electrochemical approaches, and biological synthesis are now utilized to create nanoparticles with defined shapes and sizes, enhancing their plasmonic properties.
Moreover, studies have indicated that hybrid nanoparticles, which combine noble metals with semiconductors or other materials, exhibit unique optical properties that could potentially revolutionize sensor technologies and catalysis.
Significant Breakthroughs in the Field
Key breakthroughs in the field include the discovery of new applications such as:
- Use in photothermal therapy for targeted cancer treatment
- Development of highly sensitive biosensors for disease detection
- Enhancement of light absorption in photovoltaic cells, increasing solar energy efficiency
Researchers are continuously exploring new avenues, seeking to understand and leverage the properties of plasmonic nanoparticles. The quest to unveil their full potential holds promise for significant advancements in various scientific domains.
Preamble to Plasmonic Nanoparticles
Plasmonic nanoparticles are at the forefront of materials science, especially due to their remarkable optical properties. Understanding these nanoparticles is crucial for both theoretical and practical applications in fields such as medicine, environmental science, and nanotechnology. This section focuses on their importance, including their unique characteristics that allow for applications like biosensing, spectroscopy enhancement, and photothermal therapy.
The discussion around plasmonic nanoparticles starts with defining what they are and their key features. As we explore this fascinating topic, we will also provide insights into their historical background. This context is essential to appreciate present advancements and future prospects in plasmonics.
Definition and Characteristics
Plasmonic nanoparticles are tiny metallic particles, often made from materials like gold or silver. They exhibit distinct optical properties, primarily due to surface plasmon resonance. This phenomenon occurs when conduction electrons on the surface of these metals oscillate collectively in response to incident light. This results in strong absorption and scattering of specific wavelengths of light, which is a critical feature for many applications.
The size and shape of these nanoparticles significantly influence their optical behavior. For instance, spherical nanoparticles exhibit different resonance frequencies compared to rod-shaped or triangular-shaped ones. The aspect ratio and composition also play roles in determining their electronic and optical characteristics.
In terms of their applications, one of the notable benefits of plasmonic nanoparticles is their ability to enhance local electromagnetic fields. This enhancement can lead to increased sensitivity in biosensing applications, making detection methods more efficient.
Historical Context
The study of plasmonic nanoparticles can be traced back to the early 20th century. The underlying principles of plasmonics began gaining attention through the work of scientists like Richard Feynman, who conceptualized the idea of manipulating light at the nanoscale. However, it was not until the late 1990s and early 2000s that significant advancements were made, leading to the robust field we see today.
Early applications primarily focused on biosensing and imaging, but the expansion of research revealed a broader spectrum of uses, such as in photothermal therapy and catalysis. As more researchers recognized the unique properties of these nanoparticles, the field began to grow rapidly, leading to innovative methodologies for synthesis and application.
The evolution of plasmonic nanoparticles illustrates how theoretical concepts can transform into practical tools, providing various industries with enhanced capabilities. Their versatility and functionality continue to attract significant research interest, promising a bright future for further developments.
Fundamentals of Plasmonics
The study of plasmonics is essential in understanding the behavior of plasmonic nanoparticles. These materials have unique interactions with light that enable a variety of applications. At its core, plasmonics deals with surface plasmons, which are coherent delocalized electron oscillations at the interface between a metal and a dielectric. Understanding these principles allows researchers to utilize the properties of plasmonic nanoparticles in innovative ways. This section will clarify key concepts such as Surface Plasmon Resonance, Localized Surface Plasmon Resonance, and the Electromagnetic Theory of Plasmons.
Surface Plasmon Resonance
Surface Plasmon Resonance (SPR) refers to the resonance condition that occurs when incident light hits a metal-dielectric interface at a specific angle. At this point, photons interact with surface electrons, causing them to oscillate collectively. The significance of SPR lies in its sensitivity to changes in the refractive index near the surface of the metal. As a result, SPR is widely used in sensor technology, particularly in biosensing.
Some key aspects of SPR include:
- It provides real-time detection of biological interactions.
- It enables label-free sensing in various applications.
- The technique can detect small changes in mass or refractive index, enhancing the accuracy of measurements.
Understanding SPR is vital for the effective design of optical sensors that operate with high specificity, thus expanding the horizons of biomedical diagnostics and environmental monitoring.
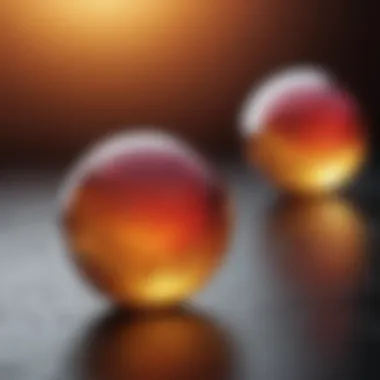
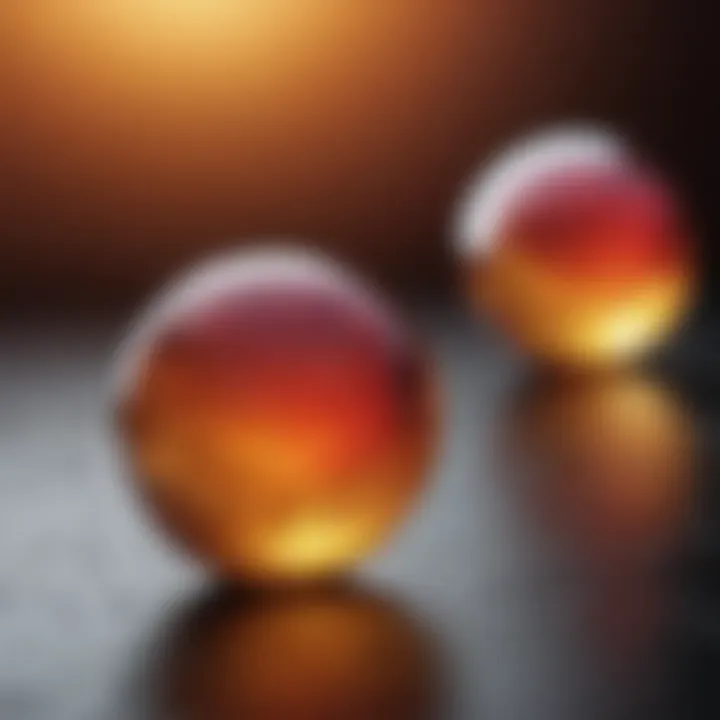
Localized Surface Plasmon Resonance
Localized Surface Plasmon Resonance (LSPR) explores how metallic nanoparticles resonate with incident light at specific wavelengths determined by their size, shape, and material composition. Unlike surface plasmon resonance that occurs at broader interfaces, LSPR occurs at discrete locations, leading to significantly enhanced electromagnetic fields in the vicinity of the nanoparticles.
Some important points regarding LSPR include:
- LSPR is strongly influenced by nanoparticle geometry.
- It is pivotal for applications in enhancing spectroscopic signals, such as in Surface-Enhanced Raman Scattering (SERS).
- This resonance allows for particle aggregation, amplifying the sensor's performance.
Characterizing LSPR can lead to advanced applications in drug delivery, imaging, and diagnostics, demonstrating its critical role in the field of plasmonics.
Electromagnetic Theory of Plasmons
The Electromagnetic Theory of Plasmons provides a theoretical framework for understanding the interaction of light with plasmonic materials. This theory integrates principles of classical electrodynamics to explain how plasmonic excitation happens and how it can be harnessed.
Key considerations in this theory include:
- The Maxwell equations describe how electromagnetic waves propagate in media, crucial for understanding plasmonic behavior.
- Non-radiative means of energy transfer are significant in materials science and applications.
- Understanding of guest-host interactions can lead to improved efficiency in optical devices.
By applying these theoretical principles, researchers can develop novel devices for sensing, imaging, and energy harvesting, further demonstrating the vast potential of plasmonic nanoparticles in modern technology.
Plasmonic nanoparticles bridge the gap between classical optics and nanotechnology, making them instrumental in advancing various scientific fields.
Plasmonic nanoparticles bridge the gap between classical optics and nanotechnology, making them instrumental in advancing various scientific fields.
Through these discussions, the fundamentals of plasmonics showcase not only their importance but also their relevance in the broader context of technology and research.
Classification of Plasmonic Nanoparticles
The classification of plasmonic nanoparticles forms a foundational component in the understanding of their diverse applications and performance. This section elucidates the two primary categories: metallic nanoparticles and alloyed/core-shell nanoparticles. Each category sheds light on the unique properties that influence their functionality in various scientific fields.
Metallic Nanoparticles
Metallic nanoparticles are one of the most well-studied and widely used types of plasmonic nanoparticles. Typically composed of noble metals such as gold and silver, these nanoparticles exhibit significant surface plasmon resonance characteristics. The size, shape, and composition of metallic nanoparticles directly affect their optical properties, resulting in tunable absorbance and scattering features.
The importance of metallic nanoparticles lies in their high conductivity and the ability to enhance local electromagnetic fields. Their unique optical features make them suitable for applications in biosensing, imaging, and drug delivery. For instance, gold nanoparticles are frequently utilized due to their biocompatibility and ease of functionalization with biomolecules. This compatibility allows for effective targeting of cells, improving the efficiency of drug delivery mechanisms.
"Metallic nanoparticles serve as significant tools in medical imaging and diagnostics due to their exceptional optical characteristics and versatility."
"Metallic nanoparticles serve as significant tools in medical imaging and diagnostics due to their exceptional optical characteristics and versatility."
Moreover, the synthesis of these nanoparticles can be achieved through several methods. Chemical reduction is a common technique employed to control size and shape during the synthesis phase. Fine-tuning these parameters can lead to optimized performance for specific applications.
Alloyed and Core-shell Nanoparticles
Alloyed and core-shell nanoparticles are innovative designs that combine the properties of different metals or materials to enhance performance. Alloyed nanoparticles often consist of two or more metals mixed at the atomic level. This amalgamation can lead to improved stability, altered conductivity, and enhanced plasmonic properties.
In contrast, core-shell nanoparticles comprise a core material surrounded by a shell of another material. Typically, the core is a noble metal while the shell may be made of a different metal or dielectric material. This structure allows for a variety of functions such as improved stability against oxidation and enhanced light absorption.
The advantage of alloyed and core-shell nanoparticles is their customizable properties, which can be tailored for specific applications. For instance, using a silver core with a gold shell can yield improved catalysis and sensing capabilities due to the synergistic effects of both metals.
In summary, the classification of plasmonic nanoparticles not only helps to understand their properties but also reveals the potential they hold for novel applications in technology and medicine. Each type of nanoparticle opens up new pathways for research and development, driving innovation in fields ranging from environmental science to nanomedicine.
Synthesis Methods of Plasmonic Nanoparticles
Synthesis methods for plasmonic nanoparticles play a crucial role in determining their optical properties, size, shape, and overall performance in various applications. A well-designed synthesis method can enhance the control over these parameters, which ultimately influence the efficacy of the nanoparticles in practical scenarios. As research and applications in plasmonics continue to grow, understanding and optimizing these methods is essential for advancing the field.
Chemical Reduction Methods
Chemical reduction methods are one of the most widely employed techniques for synthesizing plasmonic nanoparticles, especially gold and silver nanoparticles. This method typically involves reducing metal ions in a solution using a chemical agent. Common reducing agents include citric acid, sodium borohydride, and ascorbic acid. The choice of reducing agent can affect the rate of reduction and the final properties of the nanoparticles.
In this method, the size and shape of nanoparticles can be tuned by adjusting several parameters such as the concentration of metal ions, the type of reducing agent, and the reaction temperature. For instance, a higher concentration of reducing agent can result in smaller nanoparticles, while lower concentrations may produce larger structures. This flexibility allows researchers to obtain nanoparticles that meet specific requirements for various applications, including biosensing and photothermal therapy.
Physical Vapor Deposition
Physical vapor deposition (PVD) is another effective method, particularly suitable for creating thin films and coatings of plasmonic materials. PVD involves the physical transition of a material from a solid or liquid state to a vapor, which then condenses on a substrate to form a solid film. This method provides high purity and controlled thickness, which is advantageous when fabricating devices that utilize plasmonic properties.
In PVD, techniques such as thermal evaporation and sputtering are commonly used. Thermal evaporation involves heating a solid material in a vacuum until it vaporizes, whereas sputtering uses energy to eject atoms from a target material. Precision in controlling parameters during deposition is critical, as it directly impacts the film's crystallinity and morphology. This method allows for the creation of layered structures needed for sophisticated photonic devices.
Biological Synthesis Approaches
Another promising avenue for synthesizing plasmonic nanoparticles is through biological synthesis. This eco-friendly approach utilizes biological entities such as plants, bacteria, or fungi to reduce metal ions and form nanoparticles. For example, plant extracts can contain various phytochemicals that aid in the reduction process, leading to the formation of nanoparticles with specific characteristics.
The biological synthesis method offers several advantages. First, it is often less toxic compared to conventional chemical methods, making it more suitable for biomedical applications. Second, the nanoparticles synthesized biologically often exhibit unique features such as biocompatibility and stability over time. However, challenges remain, including reproducibility and scalability of the synthesis, which must be addressed for broader applications in industry.
"A clear understanding of the synthesis methods can significantly impact the performance of plasmonic nanoparticles in targeted applications."
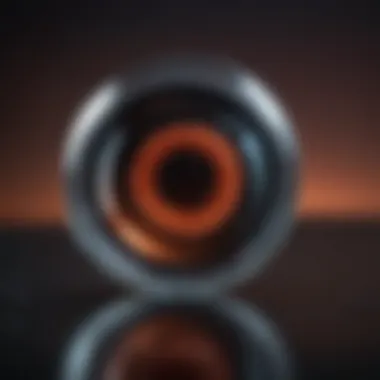
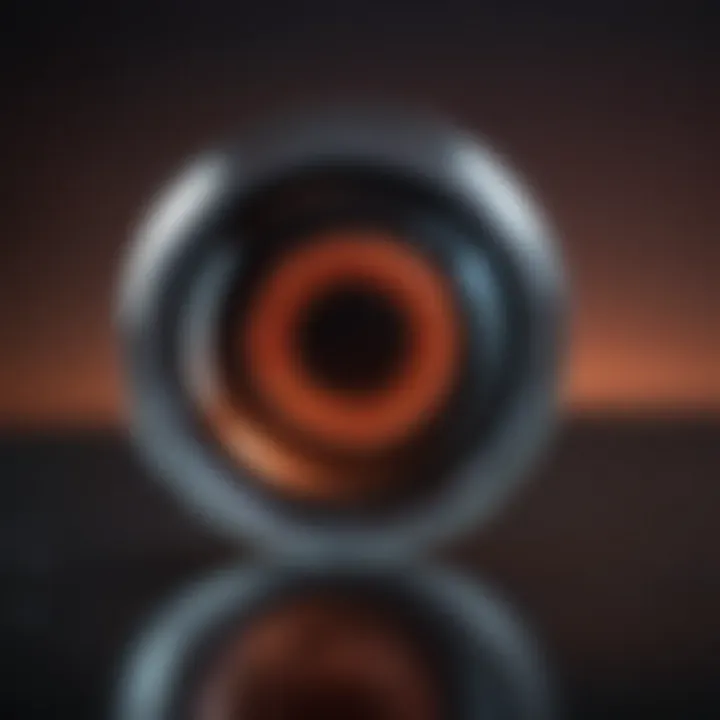
"A clear understanding of the synthesis methods can significantly impact the performance of plasmonic nanoparticles in targeted applications."
Synthesizing plasmonic nanoparticles effectively requires a thoughtful selection of methods that align with the intended application. As technological advancements continue, the evolution of synthesis techniques will likely lead to more innovative uses of plasmonic nanoparticles.
Characterization Techniques
Characterization techniques are essential in the field of plasmonic nanoparticles. These methods allow researchers to gain insight into the physical and chemical properties of these materials, enabling a better understanding of their behavior and potential applications. Accurate characterization is crucial for ensuring that plasmonic nanoparticles meet the specific requirements for use in various fields such as sensors, medicine, and environmental science. This section will explore the two predominant techniques: spectroscopy methods and electron microscopy.
Spectroscopy Methods
Spectroscopy methods are pivotal in analyzing plasmonic nanoparticles due to their capacity to study the interaction of light with matter. These techniques provide information regarding the electronic and vibrational states of the nanoparticles. They help in identifying features such as composition, size, shape and the local environment of the nanoparticles. The most commonly used spectroscopy methods include UV-Vis spectroscopy and Raman spectroscopy.
- UV-Vis Spectroscopy: This method focuses on the absorbance and transmittance of light through samples. It is particularly useful in identifying the localized surface plasmon resonance (LSPR) of nanoparticles. Changes in LSPR can indicate variations in size, shape or material composition of the nanoparticles, making it invaluable for optimizing synthesis processes.
- Raman Spectroscopy: This method detects the inelastic scattering of light, known as Raman scattering. It provides distinctive fingerprints of the molecular bonds present in a nanoparticle. Since the presence of plasmonic nanoparticles can enhance the Raman signal, this technique plays a vital role in applications like biosensing, where sensitivity is crucial.
The integration of these spectroscopy methods enhances the understanding of plasmonics by allowing researchers to correlate optical properties with the structural characteristics of nanoparticles. This correlation is essential for developing efficient plasmonic devices.
Electron Microscopy
Electron microscopy is another indispensable technique for characterizing plasmonic nanoparticles, providing critical insights into their morphology and structural features at the nanoscale. This technique can visualize particles with significantly higher resolution than optical microscopy, which is fundamental when working with nanomaterials.
- Transmission Electron Microscopy (TEM): TEM enables the observation of internal structures and allows for imaging of single nanoparticles. It provides information on crystallography, particle size, and overall morphology. The capability to analyze specific composition using techniques like energy-dispersive X-ray spectroscopy (EDX) further enriches the characterization process.
- Scanning Electron Microscopy (SEM): SEM produces three-dimensional images and can provide information on surface topography and morphology. This method is critical for studying how nanoparticles aggregate and interact with other materials or environments.
The application of electron microscopy techniques is valuable not only for academic research but also for industrial production, where quality control of nanoparticles is paramount.
Understanding the characterization of plasmonic nanoparticles is fundamental for optimizing their applications, particularly in advancing technology in healthcare and environmental science.
Understanding the characterization of plasmonic nanoparticles is fundamental for optimizing their applications, particularly in advancing technology in healthcare and environmental science.
In summary, characterization techniques such as spectroscopy methods and electron microscopy play a vital role in revealing the properties and behavior of plasmonic nanoparticles. Accurate characterization ensures that these materials can be effectively utilized in diverse applications, enhancing their contribution to science and technology.
Applications of Plasmonic Nanoparticles
The applications of plasmonic nanoparticles are vast and significant, making them an integral part of numerous scientific fields. Their unique optical properties, arising from the interactions of light with conduction electrons, are harnessed in various innovative applications. Understanding these applications reveals how plasmonic nanoparticles not only advance technology but also significantly impact practical challenges in healthcare, environmental monitoring, and analytical chemistry.
Biosensing Applications
Plasmonic nanoparticles are increasingly employed in biosensing applications. Their high sensitivity enhances the detection of biomolecules, pollutants, and pathogens. Some key advantages include:
- Increased Sensitivity: The localized surface plasmon resonance (LSPR) effect allows for the detection of low-concentration targets, providing reliable data for diagnostics.
- Real-time Monitoring: They facilitate constant observation of biochemical reactions, crucial in evaluating therapeutic efficacy or diagnosing diseases.
- Versatility: Different types of materials, such as gold and silver nanoparticles, can be tailored to target specific analytes, enhancing specificity and reducing interference.
The importance of plasmonic nanoparticles in biosensing is evident in their application in point-of-care testing. These tests often require rapid and exact results, underscoring the valuable contribution of plasmonic sensors.
Enhanced Spectroscopy Techniques
Plasmonic nanoparticles play a significant role in enhancing spectroscopic techniques. Their ability to confine light to the nanoscale enhances signal intensity, making it possible to detect even minute changes in chemical environments. Notable aspects include:
- Surface-Enhanced Raman Scattering (SERS): This technique harnesses the plasmonic effect to amplify Raman signals, allowing for detailed molecular characterization.
- Fluorescence Enhancement: Incorporating plasmonic nanoparticles can increase the efficiency of fluorescent molecules used in various applications, including tagging and imaging.
- Improved Resolution: When combined with advanced microscopy, plasmonic nanoparticles provide better resolution and contrast, which is crucial for observing nanoscale phenomena.
Enhanced spectroscopy techniques facilitated by plasmonic structures significantly contribute to fields such as nanomedicine and materials science.
Photothermal Therapy in Medicine
The potential of plasmonic nanoparticles in photothermal therapy is a significant advancement in targeted cancer treatment. By absorbing specific wavelengths of light, these nanoparticles convert light energy into heat, which can destroy cancer cells. Key points include:
- Targeted Heating: When functionalized with tumor-specific ligands, they can selectively annihilate cancerous cells while minimizing damage to surrounding healthy tissue.
- Controlled Delivery: They can be delivered via various methods, enhancing the precision of treatment.
- Combination Therapies: Photothermal therapy can be combined with other treatments, such as chemotherapy or radiotherapy, to improve overall effectiveness.
Photothermal therapy represents an emerging paradigm in oncology, with plasmonic nanoparticles paving the way for more effective and personalized treatment options.
Photonic Devices
Plasmonic nanoparticles are notable components in the development of photonic devices. These devices utilize the interplay between photons and plasmons to manipulate light on a nanoscale. Relevant considerations include:
- Waveguides: Plasmonic waveguides transport information using light, facilitating faster data transfer in communication systems.
- Sensors: Advanced optical sensors leverage plasmonic nanoparticles for enhanced detection and quantification in various environmental and clinical applications.
- Integrated Circuits: Their integration into circuits offers a promising path for creating faster and more energy-efficient electronic devices.
The innovation of photonic devices with plasmonic nanoparticles illustrates a significant advance in the field of nanotechnology, offering new solutions to longstanding technological limitations.
Plasmonic Nanoparticles in Environmental Science
Plasmonic nanoparticles hold significant promise in environmental science, primarily because of their unique optical properties and interactions with light. Their ability to enhance and modify light at the nanoscale enables various applications, including pollution detection and catalysis. These nanoparticles can play a vital role in the monitoring and remediation of environmental pollutants. In addition to this, they could potentially provide novel approaches for breaking down harmful substances in the environment. As the world grapples with increasing pollution and its consequential effects, exploring the use of plasmonic nanoparticles presents an innovative solution worth considering.
Pollution Detection
Pollution detection is critical for assessing environmental health. Traditional methods for detection often lack the sensitivity required for trace pollutants. Plasmonic nanoparticles, especially gold and silver types, can enhance the signals in spectroscopic techniques due to their surface plasmon resonance properties.
Key elements of using plasmonic nanoparticles include:
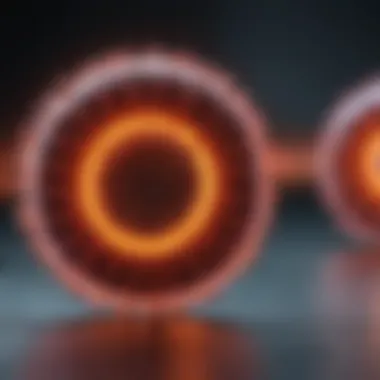
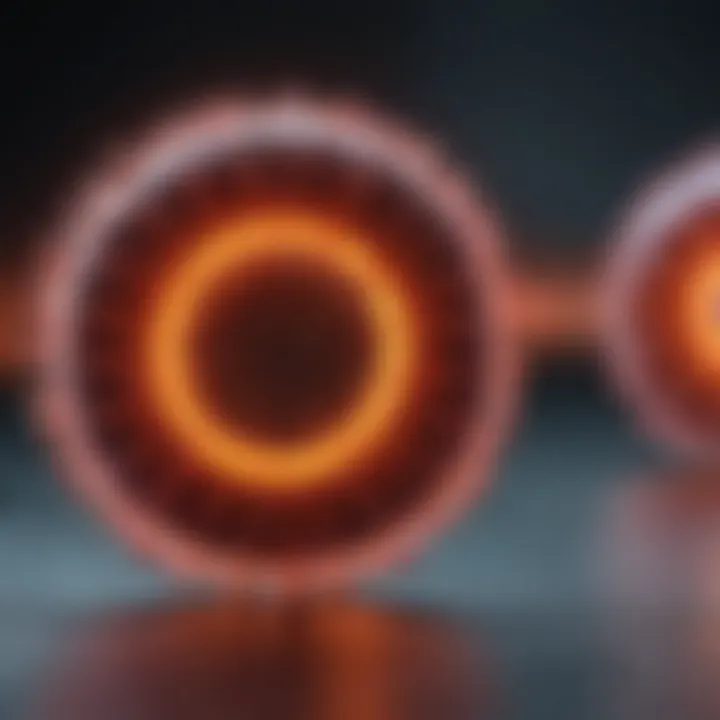
- High Sensitivity: They can detect low concentrations of pollutants, making them ideal for monitoring water sources.
- Selectivity: Tailoring the nanoparticles for specific pollutants allows for targeted detections.
- Rapid Response: Techniques utilizing these nanoparticles can provide quick results compared to traditional methods.
By binding specific chemical groups to the surface of these nanoparticles, researchers can create sensors that react to certain pollutants. When substances bind to the nanoparticles, their optical properties change. This change can be measured using techniques such as surface-enhanced Raman scattering (SERS). This makes sers a powerful tool for pollution detection, providing a non-invasive means to monitor environmental safety.
"Plasmonic nanoparticles can significantly improve the sensitivity and specificity of traditional detection methods, making them invaluable in environmental monitoring."
"Plasmonic nanoparticles can significantly improve the sensitivity and specificity of traditional detection methods, making them invaluable in environmental monitoring."
Catalysis
Catalysis involving plasmonic nanoparticles can enhance reaction rates for chemical processes. Their ability to absorb light and convert it into heat can be exploited in various applications, including the degradation of pollutants and energy conversions. These nanoparticles can act as catalysts under light, promoting reactions that would otherwise require harsher conditions.
Advantages of using plasmonic nanoparticles for catalysis include:
- Efficient Light Harnessing: Their enhanced capability to absorb light energy can drive photocatalytic reactions effectively.
- Wide Range of Applications: They can be used for breaking down organic pollutants, such as dyes, into less harmful substances.
- Potential for Renewable Energy: In energy conversion processes, they can facilitate the production of hydrogen and other clean fuels under sunlight.
Utilizing these properties in catalysis leads to eco-friendly processes, aligning well with the global push toward sustainability. Overall, exploring plasmonic nanoparticles for both pollution detection and catalysis presents a thorough understanding of their transformative potential in environmental science.
Challenges and Limitations
Understanding the challenges and limitations surrounding plasmonic nanoparticles is vital for advancing research and enhancing applications. While their unique optical properties offer great potential, several factors must be addressed to ensure their effectiveness and safety in various fields, including medicine, environmental science, and optics. This section will explore two prominent challenges: stability and aggregation issues, and toxicity concerns.
Stability and Aggregation Issues
The stability of plasmonic nanoparticles plays a crucial role in their practical applications. When these nanoparticles are synthesized, maintaining their unique properties over time is critical. Their tendency to aggregate can lead to significant changes in their optical characteristics, which can hinder their performance in real-world applications such as sensors and therapeutic agents.
Nanoparticle aggregation usually occurs due to Van der Waals forces and electrostatic interactions. Factors such as concentration, pH, and ionic strength of the surrounding medium can significantly affect their stability. For instance, when the concentration of nanoparticles increases, the likelihood of aggregation rises, resulting in a loss of plasmonic resonance.
To overcome this challenge, researchers are investigating various stabilization strategies. These include modifying the surface chemistry of nanoparticles using stabilizing agents like surfactants, polymers, and ligands. Additionally, encapsulating nanoparticles in a protective medium can minimize direct contact, thus reducing aggregation risk. Understanding these mechanisms enables the development of more stable and reliable plasmonic nanoparticles for future applications.
Toxicity Concerns
Despite the promising applications of plasmonic nanoparticles, potential toxicity raises significant concerns, particularly in biomedical fields. The interaction of nanoparticles with biological systems can lead to unintended biological responses. This can include inflammation, cytotoxicity, and even genotoxicity in certain cases.
The composition of the nanoparticles significantly influences their toxicity. For example, gold nanoparticles are generally considered biocompatible, whereas silver nanoparticles have been shown to possess higher toxicity due to their interactions with cellular components and potential release of silver ions. Thus, careful consideration of material choice is necessary when designing plasmonic nanoparticles for medical applications.
Further steps must be taken to assess the long-term impact of these materials on human health and the environment. Comprehensive toxicity studies and in vivo investigations are essential to establish safe dosage levels and predict biological responses. Only through rigorous testing can researchers ensure that plasmonic nanoparticles are used safely and effectively in various applications.
"Addressing the stability and toxicity of plasmonic nanoparticles is crucial for their successful deployment across multiple disciplines."
"Addressing the stability and toxicity of plasmonic nanoparticles is crucial for their successful deployment across multiple disciplines."
The Future of Plasmonic Nanoparticles
The future of plasmonic nanoparticles holds significant promise for advancements in multiple fields. As research evolves, a deeper understanding of their unique properties can pave the way for groundbreaking innovations. These nanoparticles offer potential benefits in science, medicine, and technology. Of particular interest are their capabilities in sensing, diagnostics, and therapy.
Innovative Research Directions
Research in plasmonic nanoparticles is expanding its horizons. A major focus is on enhancing their sensitivity for biosensing applications. By engineering nanoparticles with various shapes and sizes, scientists aim to improve detection limits in biological samples. Additionally, hybrid materials combining plasmonic nanoparticles with other nanomaterials could unlock unique functionalities. This may lead to the development of multifunctional devices, which can perform advanced tasks such as simultaneous imaging and therapy.
Another promising area of research is applications in energy conversion. Plasmonic nanoparticles can enhance light absorption, making them valuable in solar cell technology. Integrating them into solar panels could significantly improve efficiency. Furthermore, efforts to understand their role in photothermal therapy are ongoing. This technique involves using light to generate heat via plasmonic nanoparticles to selectively destroy cancer cells. The future will likely see more robust research into optimizing these processes for clinical applications.
"Innovative research will focus on harnessing the properties of plasmonic nanoparticles to develop next-generation technologies."
"Innovative research will focus on harnessing the properties of plasmonic nanoparticles to develop next-generation technologies."
Commercialization Prospects
The commercialization of plasmonic nanoparticles is still in its infancy, but the potential is immense. Industries ranging from healthcare to environmental monitoring are interested in their applications. In pharmaceutical industries, plasmonic nanoparticles can revolutionize drug delivery systems. The ability to target specific cells while minimizing side effects is a desired characteristic in modern medicine.
Moreover, sensor technologies using plasmonic nanoparticles can be commercialized to provide real-time monitoring of pathogens or pollutants. This has vast implications for public health and environmental safety. Companies focusing on these applications will likely benefit from a growing market demand.
Challenges remain. Regulatory hurdles and the need for standardized manufacturing processes could slow progress. However, as technology advances, overcoming these barriers is becoming more feasible. Overall, the commercialization landscape for plasmonic nanoparticles appears promising, with numerous opportunities for growth.
Ending
The conclusion provides an essential summary of the insights gathered throughout this article. It encapsulates the significance and broader implications of plasmonic nanoparticles in various disciplines. The exploration of their unique optical properties and applications in fields such as biosensing, photothermal therapy, and environmental science is particularly noteworthy. Understanding these aspects highlights how these nanoparticles can reshape current technologies and methodologies in scientific research and industry.
Summary of Key Findings
The key findings from the exploration of plasmonic nanoparticles can be summarized as follows:
- Unique Optical Properties: Plasmonic nanoparticles exhibit remarkable optical characteristics due to plasmon resonance, enabling applications in sensing and imaging.
- Versatile Applications: From medical therapies to environmental monitoring, their usage spans across many critical fields, showcasing their adaptability and relevance.
- Synthesis Techniques: Various synthesis methods, such as chemical reduction and biological synthesis, determine the properties and functionalities of these nanoparticles.
- Challenges: Despite their advantages, challenges such as stability, toxicity, and aggregation cannot be overlooked, necessitating careful consideration in their application.
Implications for Future Research
Future research on plasmonic nanoparticles is poised to explore several critical pathways:
- Innovative Applications: Continued investigation into new applications may lead to breakthroughs in nanomedicine and environmental remediation, expanding the scope of their utility.
- Material Development: There is a potential for developing new types of nanoparticles, improving stability and reducing toxicity concerns.
- Interdisciplinary Approaches: Collaboration across disciplines, such as chemistry, physics, and biological sciences, will likely foster novel insights and technologies.
In summary, the future of plasmonic nanoparticles is promising, filled with the potential for significant advancements in numerous fields. Research in this area should continue to focus on overcoming existing limitations while exploring innovative applications that can harness their unique properties.