Insights into Molecular Cell Biology from Alberts
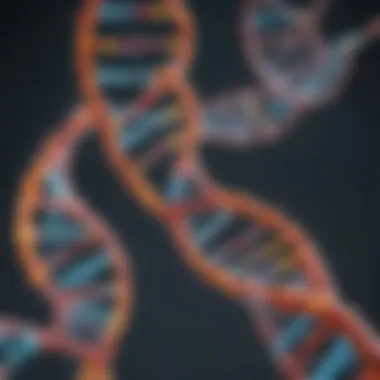
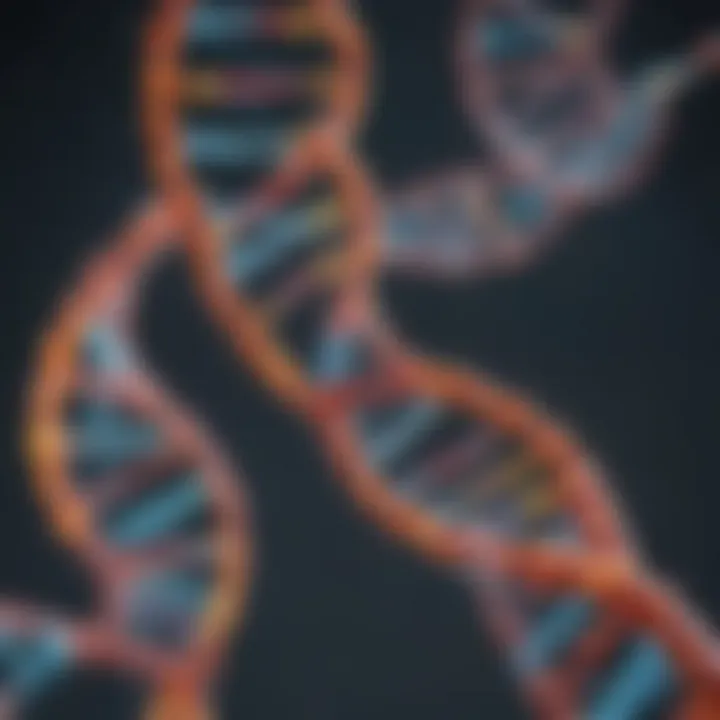
Intro
Molecular cell biology is a field that continuously evolves as we deepen our understanding of life at its most fundamental level. At the heart of this discipline lies the exploration of cells, which serve as the basic units of life, housing myriad structures critical to function and survival. The works of Bruce Alberts and his colleagues have provided invaluable insights into these complex processes, laying a groundwork for both academic inquiry and applied science.
In this article, we journey through critical concepts within molecular cell biology, weaving through the fabric of scientific principles that govern cellular mechanisms. We’ll dissect topics such as DNA replication, protein synthesis, and cellular communication, emphasizing the role they play in maintaining life. This exploration not only seeks to clarify these intricate subjects but also bridges the gap between basic understanding and advanced discussions on contemporary molecular biology.
Key Concepts
Definition of the Main Idea
The primary focus of molecular cell biology is the interplay between the molecular components of cells and their functions. This includes processes like genetic information flow, enzymatic interactions, and cellular signaling pathways. Understanding these components is crucial because they inform much of modern biology, impacting everything from medical research to biotechnological advancements.
Overview of Scientific Principles
Delving into the principles that underlie molecular biology reveals the intricacies of genetic code, enzymatic reactions, and cellular structure. Here are a few essential concepts that inform our grasp of this field:
- Genetic Information Flow: The process starts with DNA, where genetic instructions are stored. The regulation of this flow is essential for cell function.
- Proteins as Functional Molecules: Proteins play a role in nearly every cellular function, acting as catalysts, structural components, and signaling molecules. Understanding protein synthesis is key to understanding cell biology.
- Cellular Communication: Signals sent between cells can influence cellular functions and behaviors. This interplay is central to maintaining homeostasis in living organisms.
"Molecular biology provides a window into understanding the very nature of life itself; it connects the dots between the molecular and the biological."
"Molecular biology provides a window into understanding the very nature of life itself; it connects the dots between the molecular and the biological."
These principles highlight how molecules interact within the confined environment of the cell, responding to internal and external stimuli. Such understanding forms the backbone of contemporary biological inquiries.
Current Research Trends
Recent Studies and Findings
In the dynamic landscape of molecular cell biology, researchers are exploring innovative methodologies and novel discoveries. Recent studies have uncovered fascinating insights into CRISPR technology potentially allowing for precise gene editing. This technique paves the way for advancements in treating genetic diseases by targeting and altering specific genes known to cause dysfunction.
Significant Breakthroughs in the Field
A few noteworthy breakthroughs that have altered the field include:
- Discovery of RNA Interference: This process shows how certain RNA molecules can inhibit gene expression and has sparked significant interest in gene regulation.
- Insights from Structural Biology: Advances in imaging technologies, such as cryo-electron microscopy, have allowed scientists to visualize protein structures in unprecedented detail, enhancing our understanding of their functions.
- Single-Cell Sequencing Techniques: These methods have facilitated the profiling of gene expressions on a single-cell level, revealing cellular diversity within seemingly homogeneous populations.
Through such advancements, molecular cell biology continues to grow and refine the knowledge that drives both basic research and applied sciences, impacting everything from healthcare to food sources.
As we traverse through the intricacies of this field, each discovery weaves into a larger tapestry of understanding, connecting, and painting a clearer picture of the biological world.
Prelude to Molecular Cell Biology
Molecular cell biology serves as a vital discipline in the broader field of biological sciences, dissecting the intricate workings of cells at a molecular level. This journey into the microscopic realm isn't merely academic; it has profound implications for understanding life itself. Through Molecular Cell Biology, researchers unearth fundamental mechanisms that govern cellular functions, unraveling the mysteries behind everything from aging to diseases. Each process, whether it's how cells replicate their DNA or communicate with each other, ties back to molecular interactions that shape the very fabric of life.
Historical Context
To fully grasp modern molecular cell biology, one must reflect on its historical tapestry. The evolution of this field can be traced back to early observations of cells, with seminal discoveries by pioneers like Robert Hooke, who first described cells in cork in 1665. Advances flourished over the centuries, with Otto Heinrich Warburg's work on cellular respiration and the advent of microscopy providing powerful tools for exploring cellular structures. The dawn of molecular genetics in the 20th century shifted paradigms further, allowing scientists to visualize and manipulate the genetic material within cells, a leap that underpins much of today's research.
Historical milestones include the elucidation of the double helix structure of DNA by James Watson and Francis Crick in 1953, marking a pivotal moment when molecular biology intertwining genetics became possible. These foundational discoveries form a backdrop against which contemporary studies unfold, as researchers build on this legacy, addressing questions that were once deemed insurmountable.
Molecular Cell Biology Defined
Molecular cell biology can be characterized as the study of the chemical processes and substances that occur within living cells. This encompasses the understanding of various macromolecules, such as nucleic acids, proteins, carbohydrates, and lipids, which interact in complex networks to maintain life. More specifically, the field examines how these molecules contribute to cellular processes, ranging from energy production to signal transduction.
A clear definition of molecular cell biology could be encapsulated in the following aspects:
- Cell Structure: Investigating how different cellular components, like membranes, organelles, and cytoskeletons, interact to create functional units.
- Molecular Interactions: Analyzing how proteins and nucleic acids interact, how enzymes catalyze biochemical reactions, and how these processes dictate cellular responses.
- Genetic Regulation: Understanding how genes are expressed, modified, and regulated in response to environmental signals, forming the basis for how cells adapt over time.
The consequences of these studies echo throughout not just pure science but also in fields like medicine and environmental science. With the knowledge gained from molecular cell biology, scientists are better equipped to design targeted therapies for diseases such as cancer and genetic disorders, positioning the discipline at the forefront of medical advancement.
"Science is a way of thinking much more than it is a body of knowledge." - Carl Sagan
"Science is a way of thinking much more than it is a body of knowledge." - Carl Sagan
In summary, this exploration into molecular cell biology paves the way for groundbreaking research and profound insights into the biological processes that underscore all life. As we delve deeper into the mechanisms of life, we invite a deeper appreciation of the complexity, continuity, and myriad interconnections that define living organisms.
Fundamental Concepts in Cell Structure
Understanding the fundamental concepts of cell structure serves as the backbone of molecular cell biology. This knowledge not only sheds light on how cells operate but also provides insight into the intricate designs that sustain life itself. By laying a solid foundation in the components that make up cells, we can appreciate the various functions and processes that underpin biological systems. The significance of cell structure weighs heavily in various fields, enabling better advancements in medicine, biotechnology, and environmental sciences.
The Cell Theory
The cell theory is a cornerstone of biology, and grasping its principles is essential for anyone delving into molecular cell biology. This theory states that:
- All living organisms are composed of one or more cells.
- The cell is the basic unit of life.
- All cells arise from pre-existing cells.
These points, while seemingly straightforward, have monumental implications. They emphasize that cells are not just segments of organic material; they are dynamic units capable of growth, reproduction, and responding to their environment. This perspective encourages further inquiry into how cellular structures—like membranes, organelles, and cytoskeletal elements—interact harmoniously to sustain life.
Moreover, as scientists seek to understand diseases at the cellular level, the applicability of the cell theory becomes clearer. For instance, the origin of cancer lies in the aberrant behavior of cells. Understanding how normal cells function supports the identification of abnormality in cancerous cells.
Prokaryotic vs Eukaryotic Cells
The distinction between prokaryotic and eukaryotic cells is another fundamental concept in cell biology. Simply put, prokaryotic cells are the simplest form of life. They lack a nucleus and other membrane-bound organelles, making them more primitive compared to their eukaryotic counterparts.
In contrast, eukaryotic cells are more complex. They boast a well-defined nucleus that houses their genetic material and various organelles, like mitochondria, endoplasmic reticulum, and Golgi apparatus, each serving specialized functions.
Here’s a quick comparison of the two:
- Prokaryotic Cells:
- Eukaryotic Cells:
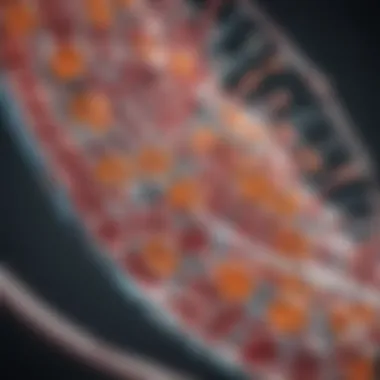
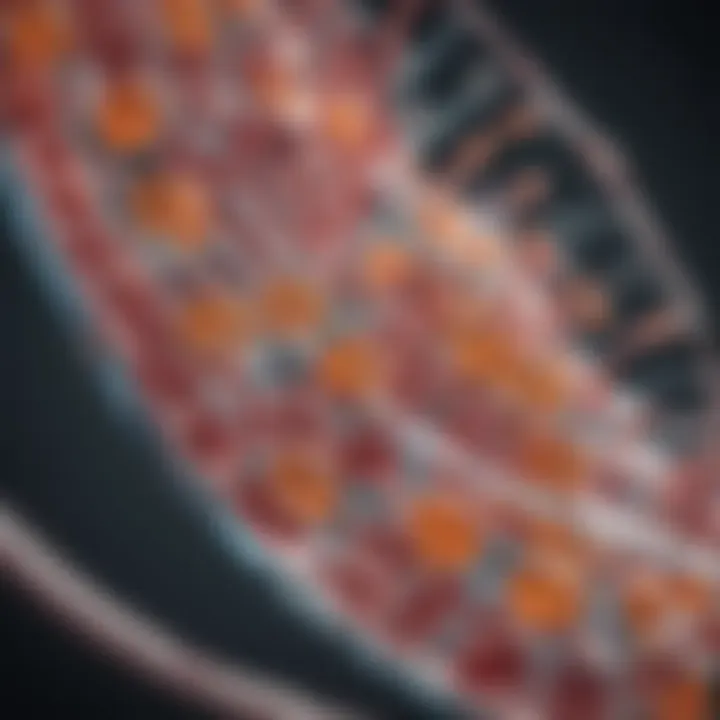
- Simple structure
- No nucleus (DNA is in the nucleoid region)
- Smaller size (generally 0.1-5.0 µm)
- Reproduce asexually mainly through binary fission
- Examples include bacteria and archaea.
- Complex structure with organized organelles
- True nucleus
- Larger size (generally 10-100 µm)
- Can reproduce sexually or asexually
- Examples include animals, plants, fungi, and protists.
The differences between these cell types are critical to understand so we can appreciate the evolutionary paths taken by different organisms. Those paths shape how species adapt and proliferate in diverse environments. Recognizing these cellular variations also plays an important role in applied sciences, such as medicine, where bacterial infections are treated differently from viral infections.
"Cells are the building blocks of life; they reveal not just structure but also the web of interactions that define biological complexity."
"Cells are the building blocks of life; they reveal not just structure but also the web of interactions that define biological complexity."
This understanding opens doors not only to the mysteries of life at a molecular level but also to potential applications, such as harnessing bacteria for bioremediation or employing yeast in biotechnology.
Molecular Components of the Cell
Molecular components are what give life its structure and function. Understanding these elements plays a crucial role in molecular cell biology, as they are the building blocks that dictate cellular behavior and interactions. This section focuses on three primary components: nucleic acids, proteins, and lipids and carbohydrates. Each of these molecular classes contributes distinctly to cellular life, providing a foundation for diverse biochemical processes that are vital for life itself. By dissecting these components, we not only observe individual functionality but also how they integrate to form complex biological systems. The interplay between these molecules underpins the life cycle of cells, influencing everything from hereditary information transfer to cellular metabolism and energy management.
Nucleic Acids
Nucleic acids, primarily DNA and RNA, serve as the blueprints of life. Their role is not merely molecular but foundational, as they encapsulate the genetic information necessary for growth, development, and functioning of all living organisms. The structure of DNA, characterized by its double helix shape, facilitates the accurate replication of genetic information, ensuring that when cells divide, each new cell receives an exact copy of the genome.
Nucleic acids undergo transcription, where a segment of DNA is copied into RNA, which then acts as a messenger to guide protein synthesis. This process enriches our understanding of gene expression — how specific genes are turned on or off in response to various signals.
Key Points about Nucleic Acids:
- Essential for genetic information storage and transfer
- DNA replication is critical for cell division
- Transcription and translation are fundamental for protein synthesis
Proteins
Proteins are the workhorses of the cell, performing a vast array of functions that are indispensable for cellular life. They act as enzymes that catalyze biochemical reactions, structural components that give shape to cells, and signaling molecules that facilitate communication between cells. Each protein's unique sequence of amino acids determines its structure and function, which is where the saying “form follows function” truly comes to life.
Moreover, proteins can undergo complex modifications after their synthesis, influencing their activity and lifespan within the cellular environment. This post-translational modification can include phosphorylation or glycosylation, impacting protein interactions and metabolic pathways significantly.
Considerations for Proteins:
- Diversity in function and structure impacts cell functionality
- Post-translational modifications add layers of regulation
- Enzymatic activity relies on precise protein folding
Lipids and Carbohydrates
Lipids and carbohydrates, while often overshadowed by nucleic acids and proteins, are equally crucial in maintaining cellular integrity and providing energy. Lipids are crucial for forming cellular membranes, enabling compartmentalization within cells, and playing a key role in signaling. They provide a hydrophobic barrier that protects cellular contents while facilitating interaction with the environment through various lipoproteins.
On the other hand, carbohydrates serve multiple roles in the cell. They are not just energy stores, but also play vital roles in cell recognition and signaling. Glycoconjugates, which are carbohydrate-protein complexes, are significant in cellular communication, influencing immune responses and the development of various cellular pathways.
Insight into Lipids and Carbohydrates:
- Membrane integrity is maintained by lipid layers
- Carbohydrates support energy production and cell interactions
- Both groups contribute to structural functions in cells
Understanding the molecular components of cells reveals not just how life functions on a microscopic level, but also how these mechanisms can be manipulated for medical and biotechnological advancements.
Understanding the molecular components of cells reveals not just how life functions on a microscopic level, but also how these mechanisms can be manipulated for medical and biotechnological advancements.
In summary, the exploration of nucleic acids, proteins, lipids, and carbohydrates unveils a rich tapestry of cellular operations. Each component plays a distinctive role, yet they harmoniously work together to maintain life, setting the stage for more complex biological interactions
The Role of DNA in Cellular Function
Understanding the role of DNA in cellular function is akin to peeling an onion. At each layer, there's something new to discover, revealing the essence of life itself. To dissect this further, one must consider DNA as not just a mere blueprint, but a dynamic participant in the orchestra of cellular processes.
The intricate structure of DNA. Its double helix shape, which is often illustrated in textbooks, hides a wealth of complexities. This architectural arrangement is not only aesthetically captivating; it provides DNA with the stability needed to store genetic information for generations. The sequence of nucleotides—adenine, thymine, cytosine, and guanine—act like a coded instruction manual, guiding the production of proteins, which are essentially the workhorses of the cell, facilitating countless biological functions.
DNA Structure and Replication
When we squarely examine DNA structure, it's crucial to acknowledge the ongoing dance of replication that allows life to perpetuate. DNA replication is a very orchestrated process, essential for cell division. The fluidity of this mechanism ensures that when cells divide, they pass on identical genetic material, which is fundamental for growth and healing.
Biologically, this requires an ensemble of enzymes like DNA polymerase. These proteins ensure fidelity during replication. Think of it as a diligent spellchecker scrutinizing each nucleotide, ensuring no errors slip through the cracks. Errors in this process could lead to significant ramifications, potentially causing diseases or developmental issues. This aspect highlights why understanding DNA replication is not merely a matter of biology; it holds implications in medicine and genetic engineering.
In summary, the beauty of DNA replication lies in its precision and necessary adaptations. As we dive into the mechanistic details, consider how this interplay of structure and function forms the underpinnings of all living organisms.
Gene Expression and Regulation
Shifting focus to gene expression, it’s like flipping a switch. Many genes sit idle, waiting for cellular signals that dictate when they will be activated. This regulation is paramount; it’s the difference between a muscle cell and a neuron. Although they share the same DNA, the selective expression of genes helps sculpt various tissues and functions.
Gene expression begins with transcription, where an enzyme called RNA polymerase reads a segment of DNA and synthesizes messenger RNA (mRNA). This mRNA carries the genetic instructions from the nucleus to the ribosomes, like a delivery service transporting essential components to the site of action. From there, translation kicks in, where ribosomes assemble proteins according to the mRNA blueprint.
However, it’s not just a one-way street. Gene regulation is multifaceted; it involves a sophisticated array of signals and feedback loops. Various proteins can enhance or repress gene expression, acting like conductors ensuring the correct genes play at the appropriate times, affecting everything from development to response in stress. Factors such as hormones and environmental stimuli can influence these pathways, making genetic regulation a responsive and adaptable mechanism.
This interplay becomes particularly relevant in understanding diseases, as aberrant gene expression can lead to conditions such as cancer, where controlled growth spirals out of hand. Therefore, delving into how genes are expressed and regulated is fundamental for both molecular biology and the larger realms of medicine and biotechnology.
"Genes do not operate in isolation; they are part of complex networks that respond to environmental cues and internal cellular states."
"Genes do not operate in isolation; they are part of complex networks that respond to environmental cues and internal cellular states."
Protein Synthesis: Translation and Beyond
In the realm of molecular cell biology, protein synthesis is paramount. This process translates the genetic code into functional proteins, vital for nearly every cellular activity. Understanding protein synthesis is not just a scholarly pursuit; it’s foundational to appreciating how cells operate, adapt, and respond to their environments.
Mechanisms of Translation
Translation is the step where ribosomes synthesize proteins from messenger RNA (mRNA). At its core, this process involves several key players, including ribosomal RNA (rRNA), transfer RNA (tRNA), and the mRNA itself. Each component has its role in ensuring that the correct amino acids are assembled into proteins.
- Initiation: The process begins when the small ribosomal subunit binds to the mRNA’s start codon. It’s somewhat akin to a conductor signaling to musicians to start playing, as the assembly line of protein production gets underway. The initiator tRNA then docks to this start codon, bringing with it the first amino acid, usually methionine.
- Elongation: After initiation, the ribosome starts to move along the mRNA. This is where things get dynamic; new tRNAs come in, each equipped with the corresponding amino acid specified by the mRNA codons. As tRNA molecules line up—like players in a line-up for a relay—amino acids are linked together by peptide bonds, forming a growing polypeptide chain. This phase continues until the ribosome reaches a stop codon on the mRNA.
- Termination: During this final stage, the ribosome encounters a stop codon, signaling that the protein is complete. Release factors bind to the ribosome, prompting the release of the newly formed polypeptide. It’s like a whistle blowing at the end of a race, signaling that the runners can finally stop.
Translation is a finely tuned sequence of events, and any hiccup can lead to misfolded proteins, impacting cell function.
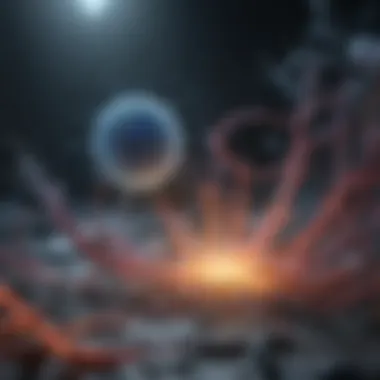
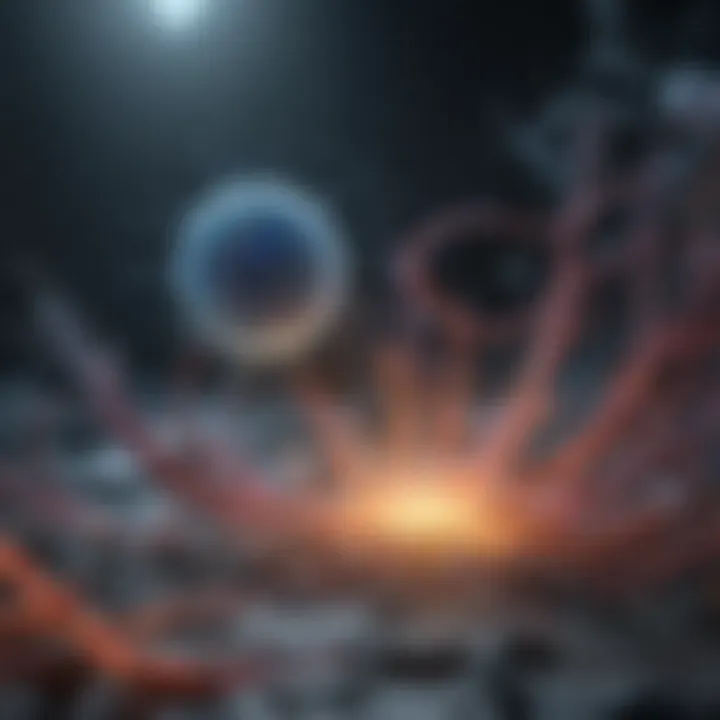
Post-Translational Modifications
After translation, proteins undergo various modifications, enhancing their functionality and versatility. The phrase "life is in the details" truly applies here. These modifications are essential, and they can lead to significant changes in protein behavior.
- Phosphorylation: Adding a phosphate group can activate or deactivate a protein, acting like a switch. For instance, kinases are enzymes that catalyze this interaction, playing a crucial role in signaling pathways.
- Glycosylation: The addition of sugar molecules alters protein stability and aids in cell-cell recognition. Think of this as putting a name tag on a protein, allowing it to be recognized by other molecules in the crowded cellular landscape.
- Ubiquitination: This modification serves as a tag for proteins destined for degradation. It's the cell's way of saying, "You've done your job; time to move on." Proteins tagged with ubiquitin are often sent to the proteasome for breakdown.
- Acetylation and Methylation: These affect the overall charge and structure of proteins, influencing their interaction with other cellular components. For example, methylation can silence genes, significantly impacting cellular responses.
"Proteins are the workhorses of the cell, performing the vast majority of functions necessary for life."
"Proteins are the workhorses of the cell, performing the vast majority of functions necessary for life."
By delving into these complex mechanisms, we gain deeper insight into the orchestration of life at the molecular level.
Cell Communication and Signaling Pathways
Cell communication and signaling pathways represent a vital backbone of molecular cell biology. These processes not only allow cells to interact and respond to their environment but also dictate how they develop and function within a multicellular organism. When cells detect signals from their surroundings or from neighboring cells, they must transmit that information effectively. This ties into the critical aspects of homeostasis, cellular growth, and response to external stimuli, forming a sophisticated language by which cells communicate with one another.
The significance of understanding cell communication cannot be overstated. A breakdown in these signaling pathways can lead to numerous disorders, including cancers, autoimmune diseases, and developmental disorders. This highlights a necessity for researchers and practitioners to engage deeply with these mechanisms. So, let’s break it down into more manageable parts, zeroing in on the different types of signaling involved in these processes and the overarching signal transduction mechanisms.
Types of Cell Signaling
Cell signaling comes in various flavors, each serving a unique purpose in the complex interaction tapestry of cellular life. Here are a few notable types:
- Autocrine Signaling: In this scenario, a cell produces signals that bind to its own receptors. This self-communication is often seen in immune cells, helping to amplify their response during an immune reaction.
- Paracrine Signaling: Unlike autocrine, paracrine signaling involves cells communicating with adjacent cells. This is notably significant in tissue growth and repair, where cells release growth factors that affect nearby cells.
- Endocrine Signaling: This is a long-distance form of communication, where hormones are released into the bloodstream to reach target organs or tissues far away. It governs numerous processes, including metabolism, growth, and mood regulation.
- Juxtacrine Signaling: Here, cells communicate through direct contact, sharing signals via molecules on their surfaces. This often occurs during embryonic development, guiding cells into their destined roles.
In summary, the different types of cell signaling create a rich ecosystem of interactions, each type tailored to specific needs and conditions of the organism.
Signal Transduction Mechanisms
Once a signal is received by a cell, it enters the realm of signal transduction—the conversion of a received signal into a functional response. This process is often multi-step and can be quite sophisticated, involving numerous components that ensure the signal is accurately relayed within the cell.
- Receptors: The first checkpoint in signaling pathways. These proteins, located on the cell membrane, bind to signaling molecules and undergo conformational changes that initiate the signaling cascade.
- Second Messengers: These are small molecules that propagate the signal inside the cell. For instance, cyclic AMP (cAMP) and calcium ions play crucial roles in amplifying the signal once a receptor is activated.
- Kinases: Enzymes that add phosphate groups to proteins, often altering the target protein's activity. This phosphorylation cascade can activate or deactivate numerous proteins in a single pathway, highlighting the intricate control the cell has.
This process is instrumental in ensuring a coordinated response to various stimuli, enabling the organism to adapt, grow, and thrive in a dynamic environment.
"Cell communication is not merely a mechanism of bio-info but can be thought of as the symphony of life playing out at the molecular level."
"Cell communication is not merely a mechanism of bio-info but can be thought of as the symphony of life playing out at the molecular level."
Cell Cycle and Division
The cell cycle is a fundamental concept in molecular cell biology, as it details the process by which cells grow, replicate, and ultimately divide. Understanding this cycle is crucial, not just for grasping how life perpetuates at the cellular level, but also for recognizing the implications it carries for growth, healing, and even cancer treatment.
The cell cycle consists of distinct phases that enable cells to prepare for division systematically. This structured approach ensures that genetic material is accurately replicated and divided, thereby maintaining genetic integrity across generations of cells. The implications of disruptions in this process can be significant, leading to various diseases, including cancer. Thus, discussing cell cycle and division is not merely academic; it's directly relevant to health and disease management, environmental adaptation, and developmental biology.
"The cell cycle is the foundation for life—understand it, and you grasp the very mechanics of biology itself."
"The cell cycle is the foundation for life—understand it, and you grasp the very mechanics of biology itself."
Phases of the Cell Cycle
The cell cycle can be broadly categorized into four key phases: G1 phase, S phase, G2 phase, and M phase. Each plays a unique role in the life of a cell:
- G1 Phase (Gap 1): In this first phase, cells grow and carry out normal metabolic processes. They assess their environment and ensure that conditions are ripe for division. During this phase, the cell’s size increases, and it synthesizes proteins necessary for DNA replication, preparing for the next stage.
- S Phase (Synthesis): The hallmark of the S phase is the replication of DNA. Each chromosome is duplicated, ensuring that when the cell divides, each daughter cell inherits an identical set of genetic material. Any errors during replication are corrected through various repair mechanisms to maintain fidelity.
- G2 Phase (Gap 2): Following DNA synthesis, the cell enters another growth phase, where it prepares for mitosis. Here, cells continue to grow and produce proteins while also checking for DNA integrity. If any damage is found, the cell can halt the cycle to fix these errors, serving as a quality control checkpoint.
- M Phase (Mitosis): This is where division occurs. Mitosis involves the separation of duplicated chromosomes into two new nuclei. It is subdivided into stages—prophase, metaphase, anaphase, and telophase—facilitating an organized distribution of genetic material.
In addition to these main phases, the cell cycles through alternate phases of G0, a resting state where cells may enter a quiescent state, not actively preparing to divide. This phase is critical for differentiating cells that may need to pause growth and participate in other functions during the organism's life.
Mechanisms of Mitosis and Meiosis
Mitosis and meiosis are two distinct methods of nuclear division, each with its purpose in the broader context of the cell cycle.
- Mitosis is responsible for somatic cell division. It ensures that two identical daughter cells are produced from the parent cell, maintaining the same chromosome number. This process is vital for growth, tissue repair, and asexual reproduction in some organisms. In each mitotic division, there’s a careful orchestration of mitotic spindle formation, chromosome alignment, and separation. This orchestration is overseen by regulatory proteins that ensure the fidelity of cell division.
- On the other hand, meiosis is a specialized type of cell division that occurs in germ cells for the purpose of producing gametes—sperms and eggs in animals. Meiosis reduces the chromosome number by half, resulting in four non-identical daughter cells. This process introduces genetic diversity through mechanisms like crossing over, where homologous chromosomes exchange segments of DNA. Such variation is essential for evolution, aiding populations in adapting to changing environments.
In summary, understanding the intricacies of the cell cycle and the mechanisms of mitosis and meiosis opens up a treasure trove of insights into how organisms grow, reproduce and adapt. It lays the groundwork not just for unraveling the mysteries of biology but also for exploring questions in health, disease, and environmental sustainability. For students, researchers, and professionals alike, these concepts are not just theoretical—they are the building blocks of life that guide scientific inquiry into the ever-evolving landscape of molecular biology.
Molecular Biology Techniques
Molecular biology techniques are foundational in the field, allowing scientists to manipulate and analyze biological molecules. With advancements in technology, these techniques have evolved to become more precise and user-friendly. Understanding these methods is crucial, as they provide insights into cellular processes and are instrumental in research and medical applications. By employing specific methodologies, researchers can uncover the minutiae of cellular mechanisms.
PCR and Gel Electrophoresis
Polymerase Chain Reaction (PCR) is a cornerstone of molecular biology. This technique enables the amplification of small segments of DNA, making it easier to study genetic material. The ability to create millions of copies of a particular DNA segment in a matter of hours is nothing short of revolutionary. PCR is widely utilized in various fields, from clinical diagnostics to forensic science.
- Steps involved in PCR:
- Denaturation: Heating the mixture to separate the strands.
- Annealing: Cooling it down to allow primers to bind to the target sequence.
- Extension: Using DNA polymerase to create new DNA strands.
This method has broad applications — it plays a critical role in identifying genetic mutations, cloning genes, and analyzing ancient DNA samples. However, knowing how to optimize reaction conditions is key to successful amplification.
On the other hand, gel electrophoresis acts as a complementary technique that allows for the separation of DNA fragments based on size. By applying an electric field to a gel matrix, DNA fragments migrate, creating distinct bands visible under UV light. This method is instrumental in:
- Verifying PCR results: Confirming that the target DNA of expected size was amplified.
- Analyzing genetic variation: Comparing DNA samples from different sources.
The synergy between PCR and gel electrophoresis exemplifies the beauty of molecular techniques: when one technique facilitates another, it leads to comprehensive insights.
The synergy between PCR and gel electrophoresis exemplifies the beauty of molecular techniques: when one technique facilitates another, it leads to comprehensive insights.
CRISPR and Genetic Engineering
CRISPR technology has taken the world of molecular biology by storm. Initially discovered as a part of the bacterial immune system, this tool allows for precise editing of the genome. Researchers can use it to cut DNA at specific locations, which can then be modified to achieve desired traits or corrections. The implications for medicine, agriculture, and biotechnology are profound.
Benefits of CRISPR include:
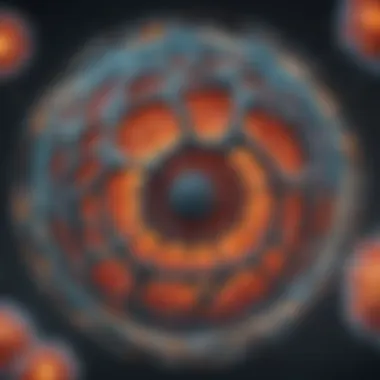
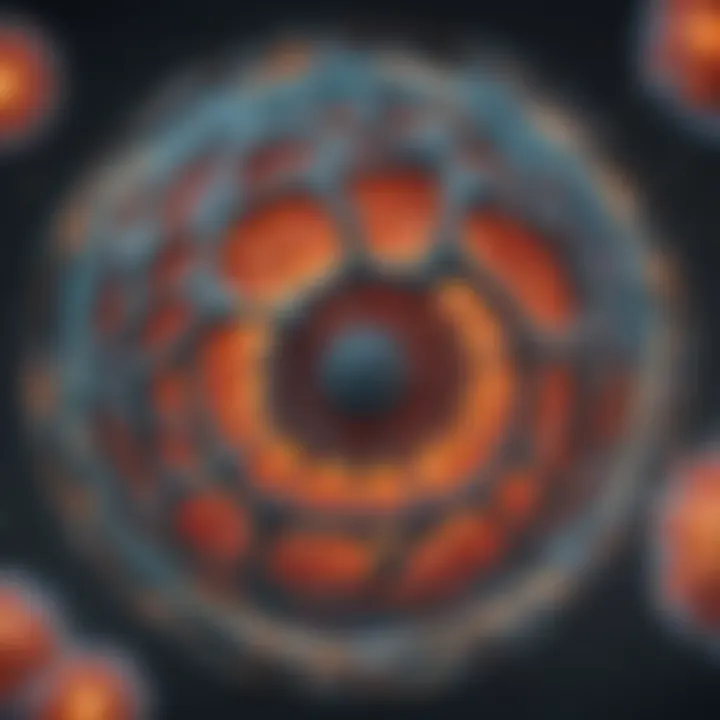
- Precision: Target specific genes without affecting others.
- Versatility: Applicable in various organisms—from plants to humans.
- Efficiency: Faster and cheaper compared to older gene-editing technologies.
Applications of CRISPR
- Gene therapy: Correcting genetic defects at the molecular level.
- Agricultural improvements: Enhancing crop resistance to diseases.
- Biomedicine: Understanding disease mechanisms to create new treatments.
However, ethical considerations surround its use, particularly regarding human germline editing. Researchers and ethicists continue to debate the implications of altering genes that can be inherited by future generations. Balancing innovation with ethical responsibility in this realm will be crucial as we march into the future of molecular biology.
In summary, molecular biology techniques like PCR and CRISPR are at the forefront of scientific discovery. They not only enhance our understanding of cellular functions but also pave the way for groundbreaking advancements in health and agriculture. As these methods become increasingly accessible, they hold the potential to transform our approach to various biological challenges.
Applications of Molecular Cell Biology
Molecular cell biology forms the backbone of many scientific fields today. Its applications stretch far beyond the laboratory benches of academia and into real-world scenarios that affect not only human health but also the environment and technological advancements. Understanding how cells operate at a molecular level unlocks doors to innovative solutions in biotechnology and medicine, as well as emphasizing the significance of preserving our ecological systems.
In the realm of biotechnology, molecular cell biology lays the groundwork for creating a myriad of products and processes. Techniques like genetic modification push the boundaries of what is possible. In fields such as agriculture, genetically modified organisms (GMOs) help create crops that are resistant to pests and diseases, which in turn can lead to food security in a changing climate. These advancements raise important discussions about our moral obligations and potential unintended consequences.
The connection between biotechnology and medicine is evident in the development of targeted therapies. For instance, understanding the molecular pathways that lead to cancer progression can lead scientists to create treatments that specifically target those pathways, minimizing side effects commonly associated with more traditional therapies.
"The challenge is not just to reduce the scariness of GMOs but to embrace the incredible possibility they bring for the betterment of society."
"The challenge is not just to reduce the scariness of GMOs but to embrace the incredible possibility they bring for the betterment of society."
However, as these fields expand, considerations surrounding ethical practices and safety must remain front and center. The fine line between innovation and ethical violations should always be tread carefully, asking ourselves: are we improving lives or risking unforeseen consequences?
Biotechnology and Medicine
The relationship between biotechnology and medicine is a true marriage of science and practicality. With a firm basis in molecular cell biology, researchers have developed novel therapies that were once considered science fiction. For example, monoclonal antibodies are engineered immune proteins that can specifically target diseased cells, providing a pathway for treatments in autoimmune disorders and cancers.
Consider also the development of CRISPR technology, a powerful tool for editing genes with precision. This technology provides scientists the ability to not only alter genes that lead to genetic disorders but also to explore possibilities like gene therapy, which could potentially cure conditions previously deemed intractable. In this case, you're not just managing symptoms; the ultimate goal is to rectify the genetic malfunction itself.
Among the benefits of these techniques are not only improved patient outcomes but also a profound understanding of underlying biological mechanisms, paving the way for future research and development.
Environmental Applications
The significance of molecular cell biology also extends to environmental science. In an era where climate change and pollution threaten biodiversity, understanding cellular responses offers insight into species adaptations and ecosystem resilience.
By applying molecular techniques, scientists can develop bioremediation strategies, using bacteria or other microorganisms to break down pollutants into less harmful substances. This not only cleans up contaminated environments but also aims to restore habitats to their natural states, ensuring the survival of various life forms.
Moreover, advancements in molecular biology enable the creation of biofuels as an alternative source of energy. By engineering organisms that produce biomass from organic materials, we may reduce reliance on fossil fuels, which have been significant contributors to greenhouse gas emissions.
Contemporary Challenges in Molecular Cell Biology
In the rapidly evolving field of molecular cell biology, researchers face a multitude of challenges that test both their scientific understanding and ethical considerations. The significance of addressing contemporary challenges cannot be overstated; it impacts how research is conducted and how findings are translated into applications. Scientists are not just piecing together the puzzles of cellular mechanisms anymore; they are also navigating complex environments that demand innovation, caution, and a deep sense of responsibility.
Understanding and Targeting Diseases
One of the foremost contemporary challenges in this discipline is the quest to understand and effectively target various diseases. With an increasing array of data available, investigators must untangle the intricate networks within cells that contribute to disease progression. For instance, cancer research exemplifies this challenge vividly. The multifaceted nature of tumors means that a one-size-fits-all approach simply won't cut it when it comes to targeted therapies.
Researchers are harnessing genetic and protein analysis to understand what drives specific cancers. This involves:
- Identifying biomarkers that can indicate disease stages or response to treatment.
- Developing personalized medicine approaches that tailor therapies to individual patient profiles.
- Assessing the role of cell signaling pathways in disease, which often dictate whether a cell proliferates, differentiates, or dies.
However, targeting diseases effectively extends beyond just understanding the biology behind them. It also involves creating treatments that are not only effective but also accessible and safe for patients. As treatments evolve, the challenge of dynamics in human genetic diversity must also be considered. This involves ensuring that interventions are effective across different populations with varied genetic backgrounds.
"As cell biology progresses, so must our understanding of its implications on human health as we tailor treatments to individuals, not just conditions."
"As cell biology progresses, so must our understanding of its implications on human health as we tailor treatments to individuals, not just conditions."
Ethical Considerations in Research
Equally significant are the ethical considerations that accompany advancements in molecular cell biology. As groundbreaking discoveries unfold, the potential for misuse or misinterpretation of data comes to the forefront. Issues like gene editing technologies, notably CRISPR, have sparked widespread discussion regarding the moral boundaries of altering genetic information. Here’s what needs to be on the radar:
- Informed Consent: Participants in studies must be thoroughly informed about how their genetic material or biological samples could be used, as well as the long-term implications of that use.
- Equity in Access: As breakthroughs occur, ensuring that all populations benefit equally from advancements in cell biology is crucial. This includes considering socio-economic factors and healthcare accessibility.
- Environmental Impact: With the intersection of biotechnology and environmental science, researchers must weigh the potential consequences of their innovations. Organisms altered for agriculture, for instance, can have unexplored effects on ecosystems.
Navigating these ethical landscapes becomes as intricate as the molecular pathways that scientists study. Researchers in the field of molecular cell biology must not only pursue knowledge but also cultivate an ethical framework that guides their investigative endeavors.
The path forward requires a confluence of scientific rigor and ethical mindfulness. Understanding and addressing these contemporary challenges is not merely a part of the scientific toolbox—it's essential to the integrity and applicability of the field.
Utilizing resources such as Britannica, Wikipedia, and the Reddit community for insights can help in further exploring these multifaceted issues.
Future Directions in Molecular Cell Biology
As we look ahead in the field of molecular cell biology, it is crucial to recognize not only the breakthroughs that have already reshaped our understanding but also the paths that are opening. This discipline is ever evolving, driven by shifts in technology, research methodologies, and an increase in interdisciplinary collaboration. Understanding these future directions not only complements our appreciation of current knowledge but also provides insight into how this knowledge will be applied to pressing challenges in healthcare, environmental science, and beyond.
Advancements in molecular cell biology have a direct impact on diverse topics, including genomics, therapeutic techniques, and ecological studies. As researchers push boundaries, they contribute to our understanding of fundamental cellular processes and their applications in specialized areas like cancer research and regenerative medicine. The benefits of following these future directions include:
- Enhanced understanding of disease mechanisms
- Development of novel therapeutic approaches
- Improvement of agricultural practices through biotechnological applications
While navigating these evolving trends, several considerations must be taken into account to ensure research remains ethical and impactful.
Emerging Research Trends
Research in molecular cell biology is venturing into exciting new directions, particularly with the increased focus on personalized medicine and precision therapies. Scientists are beginning to tailor treatments to individual genetic profiles, leading to more effective interventions. This approach takes full advantage of the wealth of data generated by large-scale genomic studies. Additionally, new tools and techniques for manipulating living cells are emerging, allowing researchers to explore cellular behavior in real time.
Some notable emerging research trends include:
- Single-cell analysis: This method allows for the examination of the individual behaviors and characteristics of cells. It’s crucial for understanding heterogeneity within populations, such as tumors, where responses to treatment can vary significantly.
- Synthetic biology: Researchers are designing and constructing new biological parts, devices, and systems. This ability could revolutionize how we approach problems ranging from sustainable energy sources to medical interventions.
- Cellular microbiology: Increased awareness of the interactions between cells and their microbial communities is guiding research towards understanding how these relationships affect health and disease.
These trends not only signify where molecular cell biology is heading but also shape the future of research itself. With more collaboration across disciplines, we can expect innovative solutions to complex biological issues.
Integrating Technology and Biology
The convergence of technology and biology is rapidly transforming the landscape of molecular cell biology. High-throughput sequencing, advanced imaging techniques, and artificial intelligence are some of the tools driving this transformation. They enable scientists to delve deeper and more effectively into cells and their processes.
- Bioinformatics: An essential component in this integration, bioinformatics combines biology with statistics and computer science to make sense of vast datasets. With the explosion of genomic data, the ability to analyze and interpret this information is critical for advancing our understanding of molecular mechanisms.
- CRISPR technology: More than just a gene-editing tool, CRISPR is facilitating insights into genetic functions and diseases with unprecedented precision. Researchers are now using this technology not just for creating genetically modified organisms but also for therapeutic applications.
- Machine learning: The application of machine learning algorithms to biological data is enabling predictions about cellular behavior and outcomes that were previously unattainable. This could lead to breakthroughs in understanding disease pathways, thus informing more targeted therapies.
Integrating these technologies allows scientists to address questions previously thought insurmountable, thus pushing the boundaries of what molecular cell biology can achieve. As we forge ahead, the melding of biological study with technological advancements will define the research landscape, ultimately paving the way for groundbreaking discoveries.